The Inner Bird
Total Page:16
File Type:pdf, Size:1020Kb
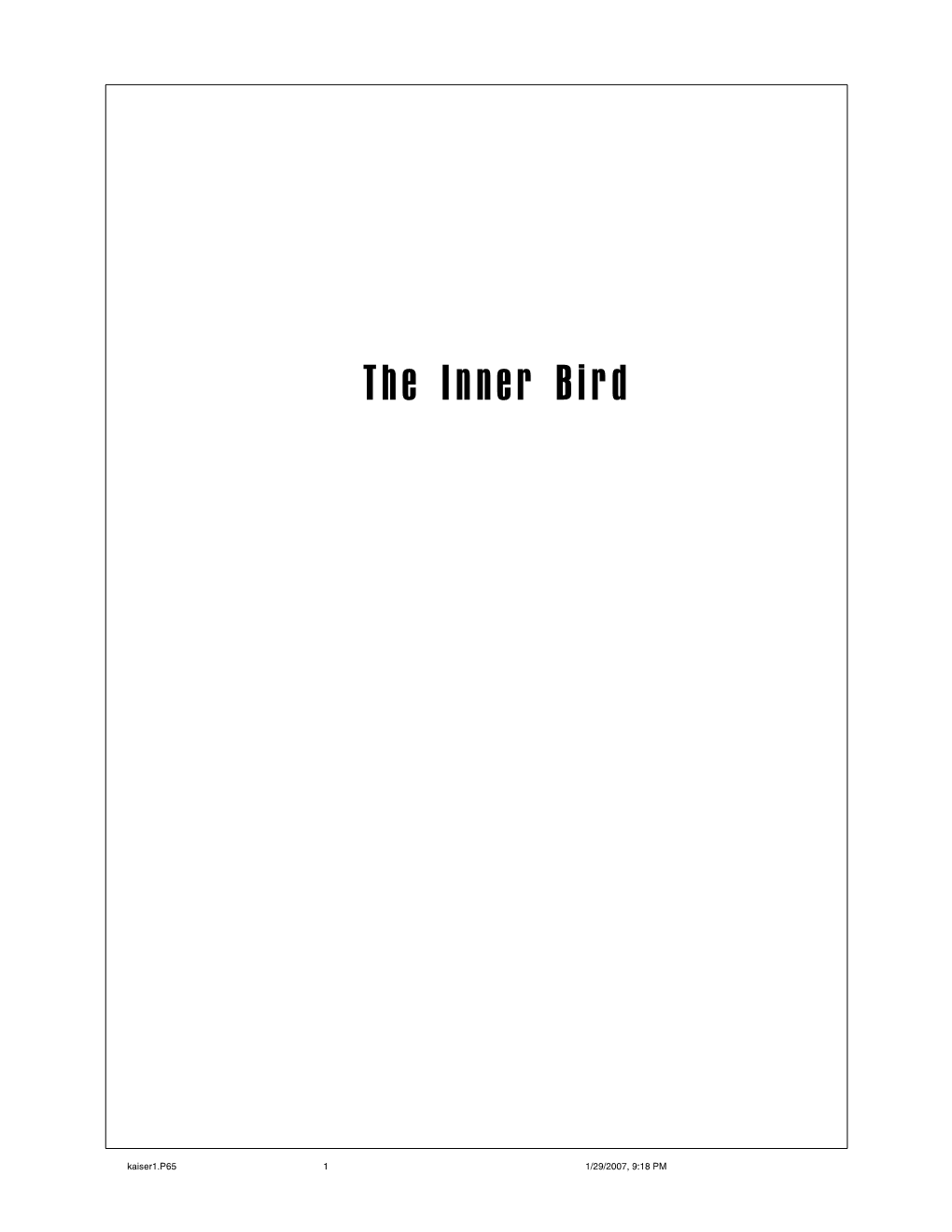
Load more
Recommended publications
-
3H?;Lnbcha Nb? 1?=L?Nm I@
3H?;LNBCHANB?1?=L?NMI@ #>O=;NIL%OC>? 1?=IH>#>CNCIH @C?F>GOM?OGILA13# 'HMC>? ;=EALIOH>'H@ILG;NCIH *?MMIHM@IL%L;>?M] >>CNCIH;F0?MIOL=?M 'HNLI>O=NCIH Unearthing the Secrets of SUE No dinosaur in the world compares to SUE—the largest, most complete, and best preserved Tyrannosaurus rex ever discovered. In May 2000, the unveiling of her 67-million-year-old skeleton at The Field Museum made global headlines. Since then, more than 16 million visitors have marveled over Chicago’s prehistoric giant. Using the story of SUE to captivate students’ imagination, the Unearthing the Secrets of SUE Educator Guide takes pre-k through eighth-grade students on an interactive exploration of SUE at The Field Museum and the scientific insights she’s providing about the world in which she lived. The lessons in this guide will engage students in the science of SUE by: • providing students unique access to SUE, the largest, most complete, and best preserved TYRANNOSAURUS REX ever discovered; • providing students with hands-on activities that enable them to investigate by making observations, developing hypotheses, questioning assumptions, testing ideas, and coming to conclusions; • introducing students to careers in science by highlighting the wide professional expertise involved in the SUE project; and • introducing students to the countless resources available to them through The Field Museum including field trips, and online research and interactive learning opportunities. How to Use this Guide • Detailed Background Information is provided to support educators in sharing the story of SUE with students. Use this information to prepare yourself and your students for learning about SUE. -
Fuzzy Simplicial Networks: a Topology-Inspired Model to Improve Task Generalization in Few-Shot Learning
Fuzzy Simplicial Networks: A Topology-Inspired Model to Improve Task Generalization in Few-shot Learning Henry Kvinge∗, Zachary New∗, Nico Courts∗y, Jung H. Lee∗, Lauren A. Phillipsz, Courtney D. Corleyz, Aaron Tuor∗, Andrew Avilaz, Nathan O. Hodasz September 24, 2020 Abstract Deep learning has shown great success in settings with massive amounts of data but has struggled when data is limited. Few-shot learning algorithms, which seek to address this limitation, are designed to generalize well to new tasks with limited data. Typically, models are evaluated on unseen classes and datasets that are defined by the same fundamental task as they are trained for (e.g. category membership). One can also ask how well a model can generalize to fundamentally different tasks within a fixed dataset (for example: moving from category membership to tasks that involve detecting object orientation or quantity). To formalize this kind of shift we define a notion of independence of tasks and identify three new sets of labels for established computer vision datasets that test a model's ability to generalize to tasks which draw on orthogonal attributes in the data. We use these datasets to investigate the failure modes of metric-based few-shot models. Based on our findings, we introduce a new few-shot model called Fuzzy Simplicial Networks (FSN) which leverages a construction from topology to more flexibly represent each class from limited data. In particular, FSN models can not only form multiple representations for a given class but can also begin to capture the low-dimensional structure which characterizes class manifolds in the encoded space of deep networks. -
Why So Many Kinds of Passerine Birds?
Letters • Why so many kinds of passerine birds? Raikow and Bledsoe (2000), in em- Slud 1976). It is unreasonable to assume the list of possible reasons for passerine bracing the null model of Slowinski that there is no underlying biological rea- success, but I would place more empha- and Guyer (1989a, 1989b), may perhaps son for this pattern and for the major sis on it than he did. be said literally to have added nothing turnover in avifaunas in the Northern It is difficult to discuss the nest-bufld- to the Kst of suggestions for why there are Hemisphere in favor of passerines after ing capabilities of passerines without re- so many species of passerine birds. When the Oligocène. sorting to anthropomorphisms such as Raikow (1986) addressed this problem Reproductive adaptations presumably "clever" or "ingenious." Is it not mar- previously and could find no key mor- made the holometabolous insects velous, however, that a highly special- phological adaptations to explain the di- (Coleóptera, Díptera, Hymenoptera, ized aerial feeder such as a cliff swallow versity of Passeriformes, the so-called Lepidoptera, and so on) the dominant (Hirundo pyrrhonota), with tiny, weak songbirds or perching birds, he despaired clade of organisms on earth. Likewise, it bill and feet, can fashion a complex nest and suggested that the problem may be appears that reproductive adaptations, out of gobs of mud fastened to a flat, only "an accident of classificatory his- not morphology, are responsible for the vertical surface? What adjective suffices tory," which brought on a storm of protest dominance of passerine birds over other to describe the nest of tailorbirds {Systematic Zoology 37: 68-76; 41: 242- orders of birds. -
Brown2009chap67.Pdf
Swifts, treeswifts, and hummingbirds (Apodiformes) Joseph W. Browna,* and David P. Mindella,b Hirundinidae, Order Passeriformes), and between the aDepartment of Ecology and Evolutionary Biology & University nectivorous hummingbirds and sunbirds (Family Nec- of Michigan Museum of Zoology, 1109 Geddes Road, University tariniidae, Order Passeriformes), the monophyletic sta- b of Michigan, Ann Arbor, MI 48109-1079, USA; Current address: tus of Apodiformes has been well supported in all of the California Academy of Sciences, 55 Concourse Drive Golden Gate major avian classiA cations since before Fürbringer (3). Park, San Francisco, CA 94118, USA *To whom correspondence should be addressed (josephwb@ A comprehensive historical review of taxonomic treat- umich.edu) ments is available (4). Recent morphological (5, 6), genetic (4, 7–12), and combined (13, 14) studies have supported the apodiform clade. Although a classiA cation based on Abstract large DNA–DNA hybridization distances (4) promoted hummingbirds and swiJ s to the rank of closely related Swifts, treeswifts, and hummingbirds constitute the Order orders (“Trochiliformes” and “Apodiformes,” respect- Apodiformes (~451 species) in the avian Superorder ively), the proposed revision does not inP uence evolu- Neoaves. The monophyletic status of this traditional avian tionary interpretations. order has been unequivocally established from genetic, One of the most robustly supported novel A ndings morphological, and combined analyses. The apodiform in recent systematic ornithology is a close relation- timetree shows that living apodiforms originated in the late ship between the nocturnal owlet-nightjars (Family Cretaceous, ~72 million years ago (Ma) with the divergence Aegothelidae, Order Caprimulgiformes) and the trad- of hummingbird and swift lineages, followed much later by itional Apodiformes. -
Onetouch 4.0 Scanned Documents
/ Chapter 2 THE FOSSIL RECORD OF BIRDS Storrs L. Olson Department of Vertebrate Zoology National Museum of Natural History Smithsonian Institution Washington, DC. I. Introduction 80 II. Archaeopteryx 85 III. Early Cretaceous Birds 87 IV. Hesperornithiformes 89 V. Ichthyornithiformes 91 VI. Other Mesozojc Birds 92 VII. Paleognathous Birds 96 A. The Problem of the Origins of Paleognathous Birds 96 B. The Fossil Record of Paleognathous Birds 104 VIII. The "Basal" Land Bird Assemblage 107 A. Opisthocomidae 109 B. Musophagidae 109 C. Cuculidae HO D. Falconidae HI E. Sagittariidae 112 F. Accipitridae 112 G. Pandionidae 114 H. Galliformes 114 1. Family Incertae Sedis Turnicidae 119 J. Columbiformes 119 K. Psittaciforines 120 L. Family Incertae Sedis Zygodactylidae 121 IX. The "Higher" Land Bird Assemblage 122 A. Coliiformes 124 B. Coraciiformes (Including Trogonidae and Galbulae) 124 C. Strigiformes 129 D. Caprimulgiformes 132 E. Apodiformes 134 F. Family Incertae Sedis Trochilidae 135 G. Order Incertae Sedis Bucerotiformes (Including Upupae) 136 H. Piciformes 138 I. Passeriformes 139 X. The Water Bird Assemblage 141 A. Gruiformes 142 B. Family Incertae Sedis Ardeidae 165 79 Avian Biology, Vol. Vlll ISBN 0-12-249408-3 80 STORES L. OLSON C. Family Incertae Sedis Podicipedidae 168 D. Charadriiformes 169 E. Anseriformes 186 F. Ciconiiformes 188 G. Pelecaniformes 192 H. Procellariiformes 208 I. Gaviiformes 212 J. Sphenisciformes 217 XI. Conclusion 217 References 218 I. Introduction Avian paleontology has long been a poor stepsister to its mammalian counterpart, a fact that may be attributed in some measure to an insufRcien- cy of qualified workers and to the absence in birds of heterodont teeth, on which the greater proportion of the fossil record of mammals is founded. -
Similarities in Body Size Distributions of Small-Bodied Flying Vertebrates
Evolutionary Ecology Research, 2004, 6: 783–797 Similarities in body size distributions of small-bodied flying vertebrates Brian A. Maurer,* James H. Brown, Tamar Dayan, Brian J. Enquist, S.K. Morgan Ernest, Elizabeth A. Hadly, John P. Haskell, David Jablonski, Kate E. Jones, Dawn M. Kaufman, S. Kathleen Lyons, Karl J. Niklas, Warren P. Porter, Kaustuv Roy, Felisa A. Smith, Bruce Tiffney and Michael R. Willig National Center for Ecological Analysis and Synthesis (NCEAS), Working Group on Body Size in Ecology and Paleoecology, 735 State Street, Suite 300, Santa Barbara, CA 93101-5504, USA ABSTRACT Since flight imposes physical constraints on the attributes of a flying organism, it is expected that the distribution of body sizes within clades of small-bodied flying vertebrates should share a similar pattern that reflects these constraints. We examined patterns in similarities of body mass distributions among five clades of small-bodied endothermic vertebrates (Passeriformes, Apodiformes + Trochiliformes, Chiroptera, Insectivora, Rodentia) to examine the extent to which these distributions are congruent among the clades that fly as opposed to those that do not fly. The body mass distributions of three clades of small-bodied flying vertebrates show significant divergence from the distributions of their sister clades. We examined two alternative hypotheses for similarities among the size frequency distributions of the five clades. The hypothesis of functional symmetry corresponds to patterns of similarity expected if body mass distributions of flying clades are constrained by similar or identical functional limitations. The hypothesis of phylogenetic symmetry corresponds to patterns of similarity expected if body mass distributions reflect phylogenetic relationships among clades. -
Beaks at the Bird Buffet
Beaks at the Bird Buffet Introduction A bird’s beak is a bony elongation of its skull that is covered in keratin, the same material that makes up your hair and fingernails. Birds use their beaks as tools. Beaks help birds gather food, groom their feathers, defend themselves, and build their nests. The shape of a bird’s beak gives us clues to figuring out its main source of food, as a bird’s beak is designed for eating a particular type of food. Raptors like owls, hawks, and vultures have grasping beaks. These beaks are hooked shaped which allows for tearing apart prey or carrion. Similar to a raptor’s beak, a cardinal’s beaks is a heavy thick beak that they use to crack seeds. Birds like a spoonbill have a long, flat, scooping beak that scoops up food like fish or insects in the water. Ducks also get their food from the water. Their flat shovel beaks allow them to scoop up fish or plants and then drain out water before swallowing. A hummingbird’s beak is long and thin, which allows it to reach into tight places for its food-like getting nectar out of a flower. For this activity, you will need a clothes pin, a spoon, chopsticks, and a craft stick - these are your beaks. A cup or bowl will serve as your bird’s stomach. Lay your “food” out on a table and select your first beak. See how much food you are able to pick up with your beak and move to your stomach in 30 seconds. -
Late Pleistocene Birds from Kingston Saltpeter Cave, Southern Appalachian Mountains, Georgia
Bull. Fla. Mus. Nat. Hist. (2005) 45(4):231-248 231 LATE PLEISTOCENE BIRDS FROM KINGSTON SALTPETER CAVE, SOUTHERN APPALACHIAN MOUNTAINS, GEORGIA David W. Steadman1 Kingston Saltpeter Cave, Bartow County, Georgia, has produced late Quaternary fossils of 38 taxa of birds. The presence of extinct species of mammals, and three radiocarbon dates on mammalian bone collagen ranging from approximately 15,000 to 12,000 Cal B.P., indicate a late Pleistocene age for this fauna, although a small portion of the fossils may be Holocene in age. The birds are dominated by forest or woodland species, especially the Ruffed Grouse (Bonasa umbellus) and Passenger Pigeon (Ectopistes migratorius). Species indicative of brushy or edge habitats, wetlands, and grasslands also are present. They include the Greater Prairie Chicken (Tympanuchus cupido, a grassland indicator) and Black-billed Magpie (Pica pica, characteristic of woody edges bordering grasslands). Both of these species reside today no closer than 1000+ km to the west (mainly northwest) of Georgia. An enigmatic owl, perhaps extinct and undescribed, is represented by two juvenile tarsometatarsi. The avifauna of Kingston Saltpeter Cave suggests that deciduous or mixed deciduous/coniferous forests and woodlands were the dominant habitats in the late Pleistocene of southernmost Appalachia, with wetlands and grasslands present as well. Key Words: Georgia; late Pleistocene; southern Appalachians; Aves; extralocal species; faunal change INTRODUCTION dates have been determined from mammal bones at Numerous late Pleistocene vertebrate faunas have been KSC. The first (10,300 ± 130 yr B.P.; Beta-12771, un- recovered from caves and other karst features of the corrected for 13C/12C; = 12,850 – 11,350 Cal B.P.) is Appalachian region (listed in Lundelius et al. -
A Synopsis of the Pre-Human Avifauna of the Mascarene Islands
– 195 – Paleornithological Research 2013 Proceed. 8th Inter nat. Meeting Society of Avian Paleontology and Evolution Ursula B. Göhlich & Andreas Kroh (Eds) A synopsis of the pre-human avifauna of the Mascarene Islands JULIAN P. HUME Bird Group, Department of Life Sciences, The Natural History Museum, Tring, UK Abstract — The isolated Mascarene Islands of Mauritius, Réunion and Rodrigues are situated in the south- western Indian Ocean. All are volcanic in origin and have never been connected to each other or any other land mass. Despite their comparatively close proximity to each other, each island differs topographically and the islands have generally distinct avifaunas. The Mascarenes remained pristine until recently, resulting in some documentation of their ecology being made before they rapidly suffered severe degradation by humans. The first major fossil discoveries were made in 1865 on Mauritius and on Rodrigues and in the late 20th century on Réunion. However, for both Mauritius and Rodrigues, the documented fossil record initially was biased toward larger, non-passerine bird species, especially the dodo Raphus cucullatus and solitaire Pezophaps solitaria. This paper provides a synopsis of the fossil Mascarene avifauna, which demonstrates that it was more diverse than previously realised. Therefore, as the islands have suffered severe anthropogenic changes and the fossil record is far from complete, any conclusions based on present avian biogeography must be viewed with caution. Key words: Mauritius, Réunion, Rodrigues, ecological history, biogeography, extinction Introduction ily described or illustrated in ships’ logs and journals, which became the source material for The Mascarene Islands of Mauritius, Réunion popular articles and books and, along with col- and Rodrigues are situated in the south-western lected specimens, enabled monographs such as Indian Ocean (Fig. -
1 Husbandry Guidelines Apodiformes Hummingbirds-Trochilidae Karen
Husbandry Guidelines Apodiformes Hummingbirds-Trochilidae Karen Krebbs, Conservation Biologist / Arizona-Sonora Desert Museum / Tucson, AZ Dave Rimlinger, Curator of Ornithology / San Diego Zoo / San Diego, CA Michael Mace, Curator of Ornithology / San Diego Wild Animal Park / Escondido, CA September, 2002 1. ACQUISITION AND ACCLIMATIZATION Sources of birds & acclimatization procedures - In the United States local species of hummingbirds can be collected with the proper permits. The Arizona-Sonora Desert Museum usually has species such as Anna's (Calypte anna), Costa's (Calypte costae), and Broad-billed (Cynanthus latirostris) for surplus each year if these species have nested in their Hummingbird Exhibit. In addition to keeping some native species, the San Diego Zoo has tried to maintain several exotic species such as Sparkling violet-ear (Colibri coruscans), Emerald (Amazilia amazilia), Oasis (Rhodopis vesper), etc. The San Diego Wild Animal Park has a large mixed species glass walk-through enclosure and has kept and produced hummingbirds over the years. All hummingbirds are on Appendix II of CITES and thus are covered under the Wild Bird Conservation Act (WBCA). An import permit from USFWS and an export permit from the country of origin must be obtained prior to the importation. Permits have been granted in the past, but currently it is difficult to find a country willing to export hummingbirds. Hummingbirds are more commonly kept in European collections, particularly private collections, and could be a source for future imports. Weighing Hummingbirds can be placed in a soft mesh bag and weighed with a spring scale. Electronic digital platform scales can also be used. A small wooden crate with a wire mesh front can also be used for weighing. -
Cranial Anatomy of Allosaurus Jimmadseni, a New Species from the Lower Part of the Morrison Formation (Upper Jurassic) of Western North America
Cranial anatomy of Allosaurus jimmadseni, a new species from the lower part of the Morrison Formation (Upper Jurassic) of Western North America Daniel J. Chure1,2,* and Mark A. Loewen3,4,* 1 Dinosaur National Monument (retired), Jensen, UT, USA 2 Independent Researcher, Jensen, UT, USA 3 Natural History Museum of Utah, University of Utah, Salt Lake City, UT, USA 4 Department of Geology and Geophysics, University of Utah, Salt Lake City, UT, USA * These authors contributed equally to this work. ABSTRACT Allosaurus is one of the best known theropod dinosaurs from the Jurassic and a crucial taxon in phylogenetic analyses. On the basis of an in-depth, firsthand study of the bulk of Allosaurus specimens housed in North American institutions, we describe here a new theropod dinosaur from the Upper Jurassic Morrison Formation of Western North America, Allosaurus jimmadseni sp. nov., based upon a remarkably complete articulated skeleton and skull and a second specimen with an articulated skull and associated skeleton. The present study also assigns several other specimens to this new species, Allosaurus jimmadseni, which is characterized by a number of autapomorphies present on the dermal skull roof and additional characters present in the postcrania. In particular, whereas the ventral margin of the jugal of Allosaurus fragilis has pronounced sigmoidal convexity, the ventral margin is virtually straight in Allosaurus jimmadseni. The paired nasals of Allosaurus jimmadseni possess bilateral, blade-like crests along the lateral margin, forming a pronounced nasolacrimal crest that is absent in Allosaurus fragilis. Submitted 20 July 2018 Accepted 31 August 2019 Subjects Paleontology, Taxonomy Published 24 January 2020 Keywords Allosaurus, Allosaurus jimmadseni, Dinosaur, Theropod, Morrison Formation, Jurassic, Corresponding author Cranial anatomy Mark A. -
Breathing and Locomotion in Birds
Breathing and locomotion in birds. A thesis submitted to the University of Manchester for the degree of Doctor of Philosophy in the Faculty of Life Sciences. 2010 Peter George Tickle Contents Abstract 4 Declaration 5 Copyright Statement 6 Author Information 7 Acknowledgements 9 Organisation of this PhD thesis 10 Chapter 1 General Introduction 13 1. Introduction 14 1.1 The Avian Respiratory System 14 1.1.1 Structure of the lung and air sacs 16 1.1.2 Airflow in the avian respiratory system 21 1.1.3 The avian aspiration pump 25 1.2 The uncinate processes in birds 29 1.2.1 Uncinate process morphology and biomechanics 32 1.3 Constraints on breathing in birds 33 1.3.1 Development 33 1.3.2 Locomotion 35 1.3.2.1 The appendicular skeleton 35 1.3.2.2 Overcoming the trade-off between breathing 36 and locomotion 1.3.2.3 Energetics of locomotion in birds 38 1.4 Evolution of the ventilatory pump in birds 41 1.5 Overview and Thesis Aims 42 2 Chapter 2 Functional significance of the uncinate processes in birds. 44 Chapter 3 Ontogenetic development of the uncinate processes in the 45 domestic turkey (Meleagris gallopavo). Chapter 4 Uncinate process length in birds scales with resting metabolic rate. 46 Chapter 5 Load carrying during locomotion in the barnacle goose (Branta 47 leucopsis): The effect of load placement and size. Chapter 6 A continuum in ventilatory mechanics from early theropods to 48 extant birds. Chapter 7 General Discussion 49 References 64 3 Abstract of a thesis by Peter George Tickle submitted to the University of Manchester for the degree of PhD in the Faculty of Life Sciences and entitled ‘Breathing and Locomotion in Birds’.