Journal of Horticulture and Forestry
Total Page:16
File Type:pdf, Size:1020Kb
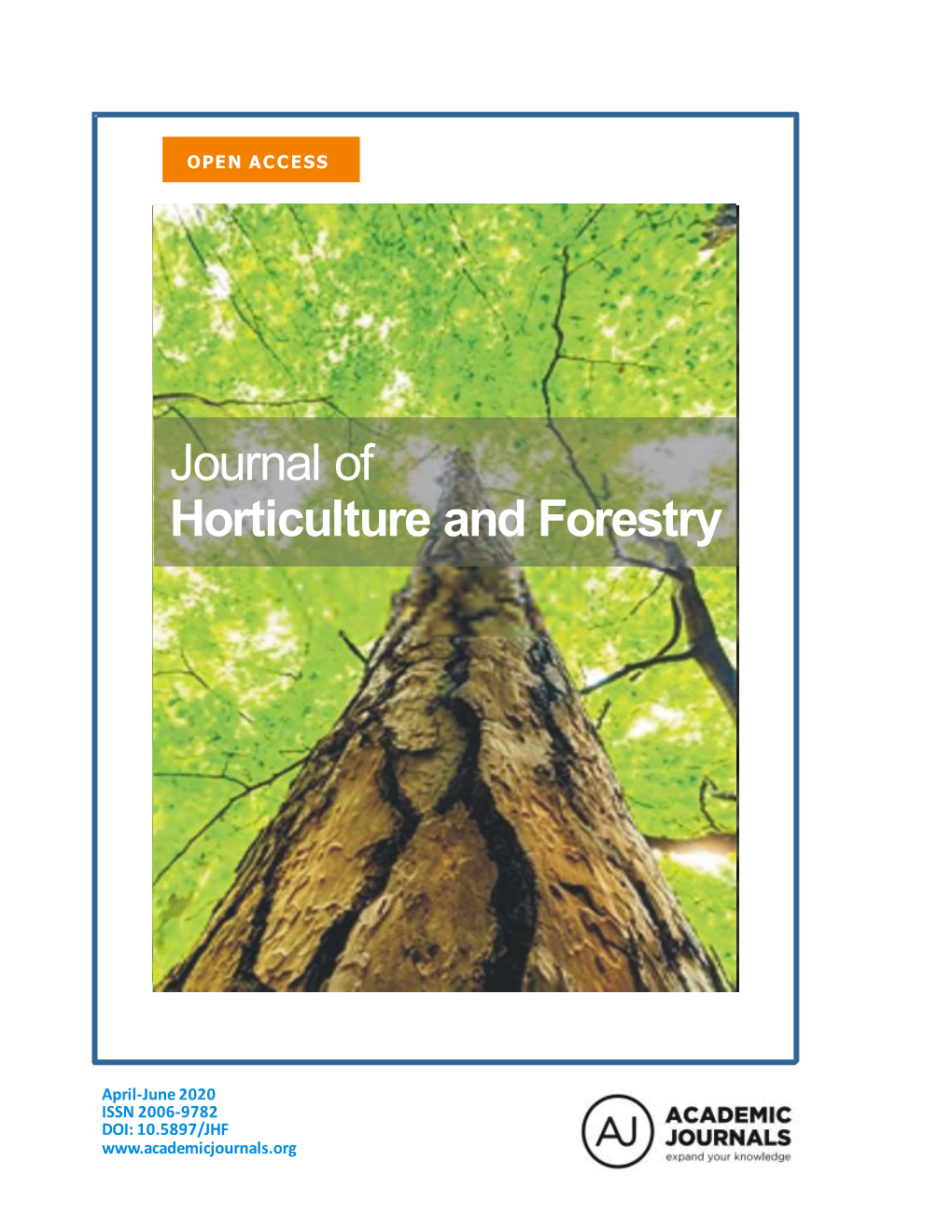
Load more
Recommended publications
-
Asteropeia Micraster
UNIVERSITÉ D'ANTANANARIVO ÉCOLE SUPÉRIEURE DES SCIENCES AGRONOMIQUES DÉPARTEMENT DES EAUX ET FORÊTS PROMOTION FITSINJO (2001-2006) MÉMOIRE DE FIN D'ÉTUDES Étude de la distribution, de l'écologie et du risque d'extinction des espèces Asteropeia micraster HALLIER , Dalbergia baroni BAKER et Dalbergia chapelieri BAILLON en vue de l'élaboration d'une stratégie de conservation de ces espèces dans la forêt littorale d'Agnalazaha (Mahabo Mananivo, Farafangana) présenté par RALAMBOMANANA-ANDRIAMAHEFA Andriamarohaja Membres du Jury Monsieur RANDRIAMBOAVONJY Jean Chrisostome Madame RAJOELISON Lalanirina Gabrielle Monsieur RABARISON Harison Monsieur RAKOTOARIVONY Fortunat Date de soutenance : 20 Novembre 2006 Résumé La forêt littorale fait partie des écosystèmes naturels les plus fragiles et sont pauvrement représentées dans le réseau des aires protégées de Madagascar. Pourtant, ce type de forêt regroupe un peu plus d'un millier d'espèces endémiques qui font la richesse floristique de l'île. La forêt littorale d'Agnalazaha, située à 50 Km au sud de Farafangana, ne fait pas exception à cette fragilité, avec d'importantes pressions anthropiques constatées, particulièrement entre 1997 et 2002. En outre, 3 espèces cibles rentrant dans la liste IUCN sont présentes sur le site étudié: Asteropeia micraster, Dalbergia baroni et Dalbergia chapelieri . Des études sur la distribution et sur l'écologie de ces espèces ont ainsi été effectuées sur le site d'Agnalazaha, afin d'évaluer les degrés des menaces auxquelles ces plantes sont confrontées, et de suggérer les mesures nécessaires à leur conservation dans la forêt d'Agnalazaha. Un travail de bibliographie, des observations sur terrain, une enquête socioéconomique et un inventaire de la forêt d'Agnalazaha ont été réalisés dans le but d'obtenir des données précises sur les espèces cibles. -
Medicinal Plants Used by Women from Agnalazaha Littoral Forest
Razafindraibe et al. Journal of Ethnobiology and Ethnomedicine 2013, 9:73 http://www.ethnobiomed.com/content/9/1/73 JOURNAL OF ETHNOBIOLOGY AND ETHNOMEDICINE RESEARCH Open Access Medicinal plants used by women from Agnalazaha littoral forest (Southeastern Madagascar) Mendrika Razafindraibe1*, Alyse R Kuhlman3, Harison Rabarison1, Vonjison Rakotoarimanana1, Charlotte Rajeriarison1, Nivo Rakotoarivelo1,2, Tabita Randrianarivony1,2, Fortunat Rakotoarivony2, Reza Ludovic2, Armand Randrianasolo3 and Rainer W Bussmann3 Abstract Background: The country of Madagascar is renowned for its high level of biodiversity and endemism, as well as the overwhelming pressures and threats placed on the natural resources by a growing population and climate change. Traditional medicine plays an important role in the daily lives of the Malagasy for various reasons including limited access to healthcare, limited markets and traditional values. The objective of this study was to assess the modern utitilization of the Agnalazaha Forest by the local population in Mahabo-Mananivo, Madagascar, for medicinal plants used by women, and to establish a list of medicinal plants used by women sourced from Agnalazaha Forest. Methods: Ethnobotanical studies were conducted over a period of five months in 2010 to determine the diversity of medicinal plants used by women in the commune of Mahabo-Mananivo. In all, 498 people were interviewed, both male and female ranging age from 15 to over 60 years old. Results: 152 medicinal plants used by local people were collected during the ethnobotanical studies. Among the recorded species, eight native species are widely used by women. These species are known for their therapeutic properties in treating placental apposition and complications during childbirth as well as tropical illnesses such as malaria, filariasis, and sexual diseases like gonorrhea and syphilis. -
Universite De Fianarantsofianarantsoa
UNIVERSITE DE FIANARANTSOFIANARANTSOA ECOLE NORMALE SUPERIEURE MEMOIRE DE RECHERCHE Pour l’obtention du CERTIFICAT D’ APTITUDE PEDAGOGIQUE DE L’ ECOLE NORMALE (C.A.P.E.N.) Filière : PHYSIQUE CHIMIE CONTRIBUTION A L’ETUDE PHYTOCHIMIQUE ET BOTANIQUE DE VOACANGA THOUARSII (Roem&Schult) (APOCYNACEAE) Présenté par RAHASINASOLO Justin Marco Soutenu le 08O ctobre 2010 devant la commission d’examen Président :Professeur RAZANAMPARANY Bruno, Examinateur :Monsieur RABEARISON Andry Hariniaina Enseignant Chercheur Rapporteur : Madame RASOANAIVO RAZAFIZANAKA Albertine, Maître de conférences SOMMAIRE Dédicace Remerciements Acronyme Liste des photos Liste des figures Liste des schémas Liste des tableaux INTRODUCTION GENERALITES Les Apocynaceae Genre Voacanga Les familles chimiques Chromatographie Propriétés pharmacologiques et biologiques des familles chimiques MATERIELS ET METHODES Matériels Méthodes adoptées SCREENING PHYTOCHIMIQUES Résultats et discussion Identification des alcaloïdes Corrélation entre usages thérapeutiques et propriétés pharmacologiques EDUCATION A L’ENVIRONNEMENT CONCLUSION GENERALE ANNEXE PREPARATION DES REACTIFS GLOSSAIRE REFFERENCES ACRONYME % : pourcent ∆ : à chaud °C : degré Celsius AcOEt : acétate d’éthyle AcOH : acide acétique Bi(NO 3) : nitrate de Bismuth CC : chromatographie sur colonne CCM : chromatographie sur couche mince CPG : chromatographie en phase gazeuse CH 2Cl 2 : dichlorométhane CHCl 3 : chloroforme CH 3COOH : acide éthanoïque CH ₃OH : méthanol cm : centimètre E.D : eau distillée Et 2O : éther éthylique EtOH -
Republique De Guinee
1 REPUBLIQUE DE GUINEE MINISTERE DES TRAVAUX PUBLICS DIRECTION NATIONALE DE ET DE L’ENVIRONNEMENT L’ENVIRONNEMENT MONOGRAPHIE NATIONALE SUR LA DIVERSITE BIOLOGIQUE GF / 6105 - 92 - 74 PNUE / GUINEE CONAKRY Novembre 1997 2 REPUBLIQUE DE GUINEE MINISTERE DES TRAVAUX PUBLICS ET DE L’ENVIRONNEMENT DIRECTION NATIONALE DE L’ENVIRONNEMENT MONOGRAPHIE NATIONALE SUR LA DIVERSITE BIOLOGIQUE UNITE NATIONALE POUR LA DIVERSITE BIOLOGIQUE COMITE DE SYNTHESE ET DE REDACTION • Mr MAADJOU BAH: Direction Nationale de l’Environnement / Coordonnateur • Dr Ahmed THIAM: Université de Conakry • Dr Ansoumane KEITA: CERESCOR • Mr Sékou SYLLA: ONG / GUINEE - ECOLOGIE • Mr Mamadou Hady BARRY DNPE / Ministère de l’Economie, du Plan et des Finances • Mr Jean LAURIAULT: Musée Canadien de la Nature 3 PRÉFACE Notre Planète abrite un ensemble impressionnant d’organismes vivants dont les espèces, la diversité génétique et les écosystèmes qu’ils constituent représentent la diversité biologique, capital biologique naturel de la terre. Cette diversité biologique présente d’importantes opportunités pour toutes les nations du monde. Elle fournit des biens et des services essentiels pour la vie et les aspirations humaines, tout en permettant aux sociétés de s’adapter aux besoins et circonstances variables. La protection de ces acquis naturels et leur exploration continue à travers la science et la technologie offrirait les moyens par lesquels les nations pourraient parvenir à un développement durable. Les valeurs économiques, éthiques, esthétiques, spirituelles, culturelles et religieuses des sociétés humaines sont une partie intégrante de cette complexe équation de protection et de conservation des acquis. Or les effets adverses des activités humaines sur la diversité biologique sont de nos jours dramatiques. -
RICHARD LAMPTEY .Pdf
KWAME NKRUMAH UNIVERSITY OF SCIENCE AND TECHNOLOGY, KUMASI BIOACTIVITY GUIDED INVESTIGATION OF THE STEM BARK AND LEAVES OF VOACANGA AFRICANA (APOCYNACEA) BY RICHARD LAMPTEY DISSERTATION SUBMITTED TO THE DEPARTMENT OF PHARMACEUTICAL CHEMISTRY OF THE FACULTY OF PHARMACY, KNUST IN PARTIAL FULFILMENT OF THE REQUIREMENTS FOR THE AWARD OF MASTER OF PHILOSOPHY DEGREE IN PHARMACEUTICAL CHEMISTRY MAY, 2016 DECLARATION I wish to declare that the work done in this thesis is my work. I further wish to declare that to the best of my knowledge, this thesis does not contain any material that has been previously published by anyone except where due acknowledgement has been made in the text. Richard Lamptey ………………………. …………………… (PG2750214) Signature Date (Student) Certified by: Dr. Joseph Adu ………………………. …………………… (Main Supervisor) Signature Date Certified by: Dr. Samuel Oppong Bekoe ………………………. …………………… (Auxillary supervisor) Signature Date Certified by: Dr. Isaac Ayensu ………………………. …………………… (Head of Department) Signature Date i ABSTRACT Plants since time immemorial have been used for their beneficial effects such as antimicrobial, antioxidant and bacterial resistance modulation. This project presents work on Voacanga africana (Apocynaceae) a local Ghanaian plant, in which the leaves and stem barks were screened for antioxidant and resistant modulatory properties. The antioxidant assay was determined using DPPH scavenging, total phenolic count and total antioxidant capacity. The antimicrobial and bacterial resistance modulation activity was determined using the broth dilution and agar well diffusion methods using S. aureus, E. coli, S. typhi, P. auregenosa, B. subtilis and Candida albicans. Further work was performed by looking at the phytochemical constituents of the extracts and isolates. The antioxidant activity as seen from the DPPH assay was concentration dependent with the methanolic extract of the stem bark showing more activity than the leaf, with Ic50 of 70 and 1605 respectively, whilst that of the standard (ascorbic acid) was 6.980. -
Savanna Fire and the Origins of the “Underground Forests” of Africa
SAVANNA FIRE AND THE ORIGINS OF THE “UNDERGROUND FORESTS” OF AFRICA Olivier Maurin1, *, T. Jonathan Davies1, 2, *, John E. Burrows3, 4, Barnabas H. Daru1, Kowiyou Yessoufou1, 5, A. Muthama Muasya6, Michelle van der Bank1 and William J. Bond6, 7 1African Centre for DNA Barcoding, Department of Botany & Plant Biotechnology, University of Johannesburg, PO Box 524 Auckland Park 2006, Johannesburg, Gauteng, South Africa; 2Department of Biology, McGill University, 1205 ave Docteur Penfield, Montreal, QC H3A 0G4, Quebec, Canada; 3Buffelskloof Herbarium, P.O. Box 710, Lydenburg, 1120, South Africa; 4Department of Plant Sciences, University of Pretoria, Private Bag X20 Hatfield 0028, Pretoria, South Africa; 5Department of Environmental Sciences, University of South Africa, Florida campus, Florida 1710, Gauteng, South Africa; 6Department of Biological Sciences and 7South African Environmental Observation Network, University of Cape Town, Rondebosch, 7701, Western Cape, South Africa *These authors contributed equally to the study Author for correspondence: T. Jonathan Davies Tel: +1 514 398 8885 Email: [email protected] Manuscript information: 5272 words (Introduction = 1242 words, Materials and Methods = 1578 words, Results = 548 words, Discussion = 1627 words, Conclusion = 205 words | 6 figures (5 color figures) | 2 Tables | 2 supporting information 1 SUMMARY 1. The origin of fire-adapted lineages is a long-standing question in ecology. Although phylogeny can provide a significant contribution to the ongoing debate, its use has been precluded by the lack of comprehensive DNA data. Here we focus on the ‘underground trees’ (= geoxyles) of southern Africa, one of the most distinctive growth forms characteristic of fire-prone savannas. 2. We placed geoxyles within the most comprehensive dated phylogeny for the regional flora comprising over 1400 woody species. -
The Status of Medicinal Plants in Central African Countries 79 5.1 Introduction 79 5.2 Burundi 80 5.3 Cameroon 81 5.4 Central African Republic 86 5.5 D
Opinions expressed in the present publication do not necessarily reflect the views of the United Nations Industrial Development Organization (UNIDO) or the Interna- tional Centre for Science and High Technology (ICS). Mention of the names of firms and commercial products does not imply endorsement by UNIDO or ICS. No use of this publication may be made for resale or for any other commercial purpose whatsoever without prior permission in writing from ICS. This is not a formal document and has been produced without formal editing. Coverpage insets include pictures of: Aloe ferox, Senna alexandrina and flowering spike of aloe ICS-UNIDO is supported by the Italian Ministry of Foreign Affairs © United Nations Industrial Development Organization and the International Centre for Science and High Technology, 2004 Earth, Environmental and Marine Sciences and Technologies ICS-UNIDO, AREA Science Park Padriciano 99, 34012 Trieste, Italy Tel.: +39-040-9228108 Fax: +39-040-9228136 E-mail: [email protected] Compendium of Medicinal and Aromatic Plants AFRICA Karan Vasisht Vishavjit Kumar Preface Africa is the world’s second largest continent after Asia, both in terms of area and population. The continent has a unique diversity of geographic and climatic factors and exceptionally rich, varied flora with an estimated 68,000 plant species, of which about 35,000 are known to be endemic. Madagascar is renowned for the highest percentage of plant species endemism in the world. Despite vast natural resources, most African countries are still struggling to pro- vide basic amenities for their people. Health facilities are deplorable and most of the population continues to rely on traditional remedies derived from medicinal plants. -
Fiche Présentation Arbre : Voacanga Thouarsii Roem. & Schult
Fiche présentation arbre : Voacanga thouarsii Statut IUCN : Roem. & Schult. (°) Non évalué (IUCN 3.1 ). (°) Nom scientifique. ↑ Utilisations Auteur © Benjamin Lisan Nom s vernaculaire s : Voacanga de Thouars (Fr), Wild frangipani (En), Mlind aziwa (Sw) Noms vernaculaires malgaches : Kaboka, Voakanga ou Voacanga, Montaka, Folitra, Montagana … (Sources : A) Prota database, www.prota4u.org , B) CD-ROM Plantes médicinales de Madagascar , Pierre Boiteau et Lucile Allorge-Boiteau, cité dans la partie « bibliographie » de cette fiche, C) Page Internet « Les plantes médicinales malgaches et leurs utilisations », cité dans la partie « bibliographie » de cette fiche). Nom s commun s : Nom s commerciaux : Synonyme (s) : Orchipeda thouarsii (Roem. & Schult.) Baron (1905) (Source : Prota database, www.prota4u.org ). Distribution, r épartition et rég ions géographique s : Image ou photo de l’Arbre L’espèce « Voacanga thouarsii » ne se trouve qu’à Madagascar. Note : Cette espèce ne doit pas être confondue avec l’espèce « Voacanga africana 1 »2 3. Arbre. Source : http://tanisiaina.com/NM_APOCYNACEAE_ Page2.htm Latitudes géographiques (°N/ °S): Fourchette d’altitudes : 0 à 600 (1600) m. Origine : Afrique tropicale et Madagascar. Régions d'introduction connues : ? Classification classique Classif ication phylogénétique Caractéristiques physiques / dimensions Règne : Plantae Clade : Angiospermes Hauteur maximale arbre : 15 (20) m Sous -règne : Tracheobionta Clade : Dicotylédones vraies Hauteur maximale tronc : m Division : Dicotylédones vraies Clade : Astéridées ∅∅∅ adulte à hauteur d’homme (1,3m) : 40(80) cm Classe : Astéridées Clade : Lamiidées Densité : ~ kg/m3 (à ans et à % humidité ) Sous -classe : Lamiidées Ordre : Gentianales Pouvo ir calorifique : kcal/kg Ordre : Gentianales Famille : Apocynaceae Durée de vie : ? Famille : Apocynaceae Sous -famille : Rauvolfioideae Genre : Voacanga Espèce : Voacanga thouarsii Tribu : Tabermontantaneae Nom binominal : Voacanga Groupe : Feuillu. -
Plant Conservation in Communities on the Chimanimani Footslopes, Mozambique
Darwin Initiative Award 2380: Balancing Conservation and Livelihoods in the Chimanimani Forest Belt, Mozambique PLANT CONSERVATION IN COMMUNITIES ON THE CHIMANIMANI FOOTSLOPES, MOZAMBIQUE April 2016 Jonathan Timberlake, Iain Darbyshire, Martin Cheek, Aurelio Banze, Valdemar Fijamo, João Massunde, Hercilia Chipanga and Daglasse Muassinar Plant conservation on the Chimanimani footslopes, Mozambique, page 2 of 69 Front cover: Rio Tave grasslands, Mpunga community (TT). Frontispiece: Team at Thekeza homestead, Zomba (TT, top); Team collecting plants, Mpunga (TT, middle L); Woodland clearance for maize, Mahate (JT, middle R); Fruit of Pterocarpus angolensis, Mpunga (MC, bottom L); Forest stream, Maronga (JT, bottom R). Photo credits: JT – Jonathan Timberlake TT – Tom Timberlake MC – Martin Cheek ID ‒ Iain Darbyshire Suggested citation: Timberlake, J.R., Darbyshire, I., Cheek, M., Banze, A., Fijamo, V., Massunde, J., Chipanga H. and Muassinar, D. (2016). Plant conservation in communities on the Chimanimani footslopes, Mozambique. Report produced under the Darwin Initiative Award 2380. Royal Botanic Gardens, Kew, London. 69 pp. Plant conservation on the Chimanimani footslopes, Mozambique, page 3 of 69 LIST OF CONTENTS LIST OF CONTENTS ............................................................................................................... 3 SUMMARY ............................................................................................................................... 4 1. INTRODUCTION ................................................................................................................ -
Original Article a Novel Phylogenetic Regionalization of Phytogeographic
1 Article type: Original Article A novel phylogenetic regionalization of phytogeographic zones of southern Africa reveals their hidden evolutionary affinities Barnabas H. Daru1,2,*, Michelle van der Bank1, Olivier Maurin1, Kowiyou Yessoufou1,3, Hanno Schaefer4, Jasper A. Slingsby5,6, and T. Jonathan Davies1,7 1African Centre for DNA Barcoding, University of Johannesburg, APK Campus, PO Box 524, Auckland Park, 2006, Johannesburg, South Africa 2Department of Plant Science, University of Pretoria, Private Bag X20, Hatfield 0028, South Africa 3Department of Environmental Sciences, University of South Africa, Florida Campus, Florida 1710, South Africa 4Technische Universität München, Plant Biodiversity Research, Emil-Ramann Strasse 2, 85354 Freising, Germany 5Fynbos Node, South African Environmental Observation Network, Private Bag X7, 7735, Rhodes Drive, Newlands, South Africa 6Department of Biological Sciences, University of Cape Town, Private Bag X3, Rondebosch 7701, South Africa 7Department of Biology, McGill University, Montreal, QC H3A 0G4, Canada *Correspondence: Barnabas H. Daru, Department of Plant Science, University of Pretoria, Private Bag X20, Hatfield 0028, South Africa. Journal of Biogeography 2 Email: [email protected] Running header: Phylogenetic regionalization of vegetation types Manuscript information: 266 words in the Abstract, 5060 words in manuscript, 78 literature citations, 22 text pages, 4 figures, 1 table, 4 supplemental figures, and 3 supplemental tables. Total word count (inclusive of abstract, text and references) = 7407. Journal of Biogeography 3 Abstract Aim: Whilst existing bioregional classification schemes often consider the compositional affinities within regional biotas, they do not typically incorporate phylogenetic information explicitly. Because phylogeny captures information on the evolutionary history of taxa, it provides a powerful tool for delineating biogeographic boundaries and for establishing relationships among them. -
African Medicinal Plants: Setting Priorities at the Interface Between Conservation and Primary Health Care
PEOPLE AND PLANTS WORKING1 PAPER - MARCH 1993 This series of working papers is intended to provide information and to generate fruitful discussion on key issues African in the sustainable and equitable use medicinal plants of plant resources. Please Setting priorities send comments at the interface between conservation on this paper and primary healthcare and suggestions A.B. Cunningham for future issues to People and Plants Initiative, Division of Ecological Sciences, UNESCO, 7 Place de Fontenoy, 75352 Paris CEDEX 07 SP, France. The designations employed and the presentation of material throughout this publication do not imply the expression of any opinion whatsoever on the part of UNESCO concerning the legal status of any country, territory, city, or area of its authorities, or concerning the delimitation of its frontiers or boundaries. The opinions expressed in this paper are entirely those of the author and do not commit any Organization. Author's address: A.B. Cunningham 84 Watkins Street - White Gum Valley, Fremantle 6162 Western Australia - Australia Published in 1993 by the United Nations Educational, Scientific and Cultural Organization, 7 Place de Fontenoy, 75352 Paris CEDEX 07 SP. Printed by UNESCO Presse on chlorine-free recycled paper. Series editor: Alison Semple Design and layout: Ivette Fabbri Suggested citation: Cunningham, A.B. (1993). African medicinal plants: setting priorities at the interface between conservation and primary health care. People and Plants working paper 1. Paris. UNESCO. African medicinal plants SETTING PRIORITIES AT THE INTERFACE BETWEEN CONSERVATION AND PRIMARY HEALTHCARE Abstract Sustainable management of traditional medici- use of bark, roots or whole plants used as herbal nal plant resources is important, not only medicines is possible, the high levels of money because of their value as a potential source of and manpower required for intensive manage- new drugs, but due to reliance on traditional ment of slow growing species in multiple- medicinal plants for health. -
Full Text Article
MEP Notes 22-25 Mada Candollea 67-1_. 23.07.12 11:14 Page137 Notes on the flora of Madagascar, 22-25 Martin W. Callmander, Peter B. Phillipson & Laurent Gautier (ed.) Abstract Résumé CALLMANDER, M. W., P. B. PHILLIPSON & L. GAUTIER (ed.) (2012). CALLMANDER, M. W., P. B. PHILLIPSON & L. GAUTIER (ed.) (2012). Notes on the flora of Madagascar, 22-25. Candollea 67: 137-151. In French Notes sur la flore de Madagascar, 22-25. Candollea 67: 137-151. En français and English, English and French abstracts. et anglais, résumés anglais et français. Ongoing research on Madagascar’s flora is revealing numerous Les recherches en cours sur la flore de Madagascar révèlent taxonomic novelties and nomenclatural inconsistencies, and de nombreuses nouveautés taxonomiques, des problèmes de providing new data on species distribution. This is the fourth nomenclature et de nouvelles données sur la distribution des set of notes in a series that aims to provide the botanical com- espèces. Cette publication est la quatrième d’une série de notes munity working on the flora of Madagascar an opportunity to destinées à donner à la communauté botanique internationale publish short communications on these topics, and comprises travaillant sur Madagascar la possibilité de publier de courtes four notes. contributions traitant de ces aspects et comprend quatre notes. – Note 22. Description of the fruit of Tabernaemontana capuronii – Note 22. Description du fruit de Tabernaemontana capuronii Leeuwenb. (Apocynaceae), 40 ans après la découverte de Leeuwenb. (Apocynaceae), 40 years after the discovery of this l’espèce, par Lucile Allorge & Adolphe Lehavana. Description species, by Lucile Allorge & Adolphe Lehavana.