WIND and AVERAGE AIRFLOW at DIFFERENT ESTONIAN COASTAL STATIONS DURING 1966- 2005 Master's Thesis
Total Page:16
File Type:pdf, Size:1020Kb
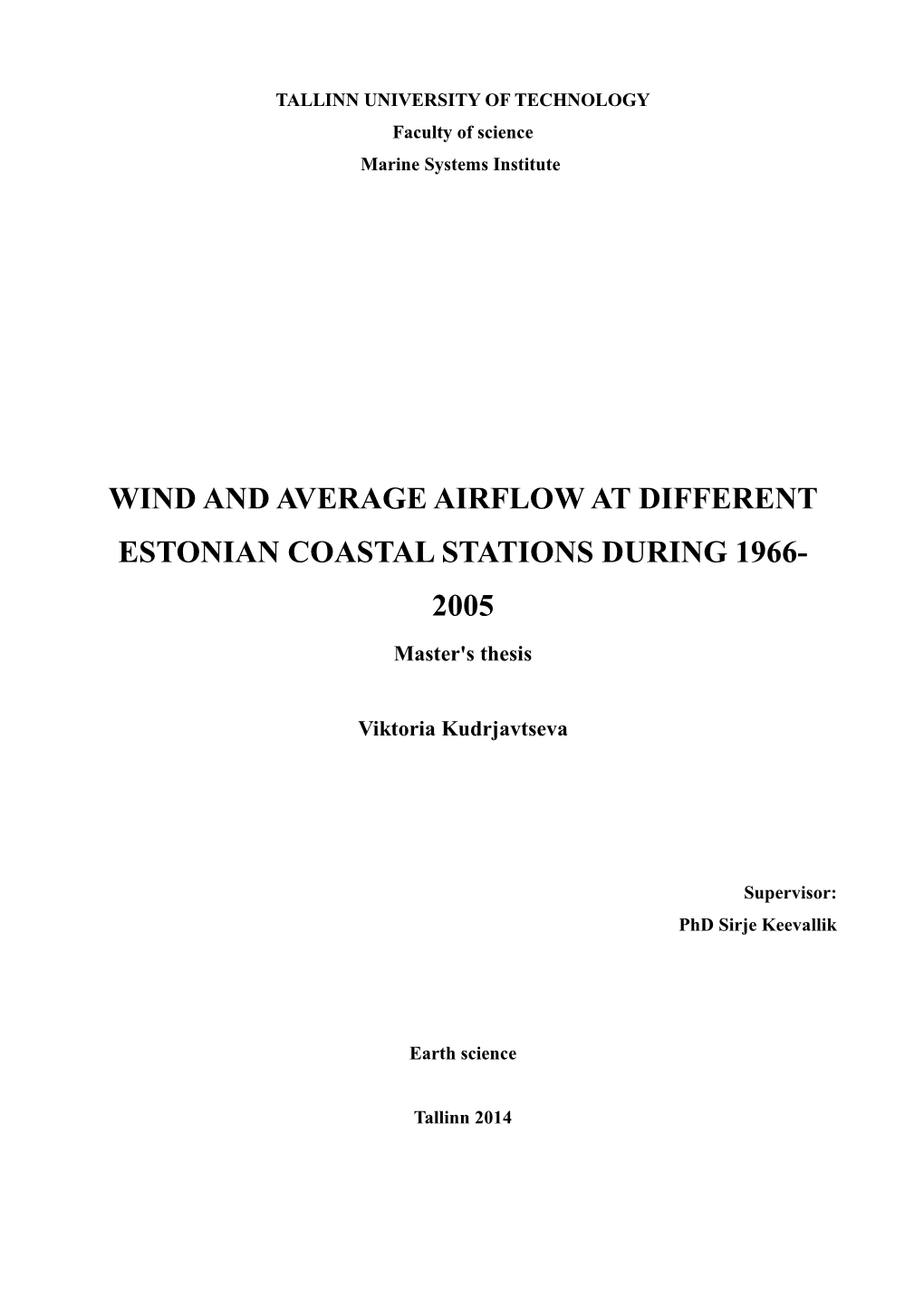
Load more
Recommended publications
-
The Transformation of Arachne Into a Spider.Pdf
Name: Class: The Transformation of Arachne into a Spider By Ovid From Metamorphoses (Book Vi) 8 A.D. Ovid (43 B.C.-17 A.D.) was a Roman poet well-known for his elaborate prose and fantastical imagery. Ovid was similar to his literary contemporary, Virgil, in that both authors played a part in reinventing classical poetry and mythology for Roman culture. Metamorphoses, one of Ovid’s most-read works, consists of a series of short stories and epic poems whose mythological characters undergo transformation in some way or another. As you read, take notes on Ovid’s choice of figurative language and imagery. [1] Pallas,1 attending to the Muse's2 song, Approv'd the just resentment of their wrong; And thus reflects: While tamely3 I commend Those who their injur'd deities4 defend, [5] My own divinity affronted stands, And calls aloud for justice at my hands; Then takes the hint, asham'd to lag behind, And on Arachne' bends her vengeful mind; One at the loom5 so excellently skill'd, [10] That to the Goddess6 she refus'd to yield. Low was her birth, and small her native town, She from her art alone obtain'd renown. Idmon, her father, made it his employ, "Linen Weaving" is licensed under CC BY-ND 2.0. To give the spungy fleece7 a purple dye: [15] Of vulgar strain her mother, lately dead, With her own rank had been content to wed; Yet she their daughter, tho' her time was spent In a small hamlet,8 and of mean descent, Thro' the great towns of Lydia gain'd a name, [20] And fill'd the neighb'ring countries with her fame. -
Oracles of Dionysos in Ancient Thrace
UDK 398.3:25(398 Trakija) Haemus Journal, vol. 2, 2013, 61-70, ISSN: 1857- 841161 Oracles of Dionysos in Ancient Thrace Jordan Iliev Independent Researcher, Sofia, BG [email protected] Abstract: The focus of the article is the available information about the oracles of Dionysos in ancient Thrace. After a careful analysis of the currently know sources the author states that in the ancient Thracian lands there was not only one or two (as most modern scholars assume) but several oracles of Dionysos. The author proves this point by giving a special attention to some details in the ancient information, such as the localization of the different oracles, the features of the mantic session, the officials etc. In conclusion is summed up that although the provided by the ancient writers’ more or less detailed information, oracles of Dionysos in Thrace are not yet localized. Key words: Ancient divination, Dionysos, oracles, Thrace. Dionysos, the famous ancient Greek god of vines and wine, was strongly connected with the Thracian lands.1 In the following lines is paid attention to only one aspect of this connection – the available information about his oracles by the Thracians. The essential sources on this issue are only a few passages in the ancient literature, presented below.2 They are repeatedly discussed by the modern scholars, which bring together all sources with only one or two oracles of that god in Thrace.3 However, here is suggested a new interpretation, based on a careful analysis of the ancient sources with a special attention over some important details neglected by the researchers, such as the localization of the different oracles, features of the mantic session, the officials etc. -
Denver Graninger, Late Argeads in Thrace: Religious Perspectives
The Ancient History Bulletin VOLUME THIRTY-ONE: 2017 NUMBERS 3-4 Edited by: Timothy Howe òEdward Anson ò Michael Fronda David Hollander òJoseph Roisman ò John Vanderspoel Pat Wheatley ò Sabine Müller òAlex McAuley Catalina Balmacedaò Charlotte Dunn ISSN 0835-3638 ANCIENT HISTORY BULLETIN Volume 31 (2017) Numbers 3-4 Edited by: Edward Anson, Catalina Balmaceda, Michael Fronda, David Hollander, Alex McAuley, Sabine Müller, Joseph Roisman, John Vanderspoel, Pat Wheatley Senior Editor: Timothy Howe Assistant Editor: Charlotte Dunn Editorial correspondents Elizabeth Baynham, Hugh Bowden, Franca Landucci Gattinoni, Alexander Meeus, Kurt Raaflaub, P.J. Rhodes, Robert Rollinger, Victor Alonso Troncoso Contents of volume thirty-one Numbers 3-4 70 Timothy Doran, Nabis of Sparta: Heir to Agis IV and Kleomenes III? 92 Christopher Tuplin, The Great King, his god(s) and intimations of divinity. The Achaemenid hinterland of ruler cult? 112 Michael Kleu, Philip V, the Selci-Hoard and the supposed building of a Macedonian fleet in Lissus 120 Denver Graninger, Late Argeads in Thrace: Religious Perspectives NOTES TO CONTRIBUTORS AND SUBSCRIBERS The Ancient History Bulletin was founded in 1987 by Waldemar Heckel, Brian Lavelle, and John Vanderspoel. The board of editorial correspondents consists of Elizabeth Baynham (University of Newcastle), Hugh Bowden (Kings College, London), Franca Landucci Gattinoni (Università Cattolica, Milan), Alexander Meeus (University of Leuven), Kurt Raaflaub (Brown University), P.J. Rhodes (Durham University), Robert Rollinger (Universität Innsbruck), Victor Alonso Troncoso (Universidade da Coruña) AHB is currently edited by: Timothy Howe (Senior Editor: [email protected]), Edward Anson, Catalina Balmaceda, Michael Fronda, David Hollander, Alex McAuley, Sabine Müller, Joseph Roisman, John Vanderspoel and Pat Wheatley. -
1 Name 2 Zeus in Myth
Zeus For other uses, see Zeus (disambiguation). Zeus (English pronunciation: /ˈzjuːs/[3] ZEWS); Ancient Greek Ζεύς Zeús, pronounced [zdeǔ̯s] in Classical Attic; Modern Greek: Δίας Días pronounced [ˈði.as]) is the god of sky and thunder and the ruler of the Olympians of Mount Olympus. The name Zeus is cognate with the first element of Roman Jupiter, and Zeus and Jupiter became closely identified with each other. Zeus is the child of Cronus and Rhea, and the youngest of his siblings. In most traditions he is married to Hera, although, at the oracle of Dodona, his consort The Chariot of Zeus, from an 1879 Stories from the Greek is Dione: according to the Iliad, he is the father of Tragedians by Alfred Church. Aphrodite by Dione.[4] He is known for his erotic es- capades. These resulted in many godly and heroic offspring, including Athena, Apollo, Artemis, Hermes, the Proto-Indo-European god of the daytime sky, also [10][11] Persephone (by Demeter), Dionysus, Perseus, Heracles, called *Dyeus ph2tēr (“Sky Father”). The god is Helen of Troy, Minos, and the Muses (by Mnemosyne); known under this name in the Rigveda (Vedic San- by Hera, he is usually said to have fathered Ares, Hebe skrit Dyaus/Dyaus Pita), Latin (compare Jupiter, from and Hephaestus.[5] Iuppiter, deriving from the Proto-Indo-European voca- [12] tive *dyeu-ph2tēr), deriving from the root *dyeu- As Walter Burkert points out in his book, Greek Religion, (“to shine”, and in its many derivatives, “sky, heaven, “Even the gods who are not his natural children address [10] [6] god”). -
Zeus in the Greek Mysteries) and Was Thought of As the Personification of Cyclic Law, the Causal Power of Expansion, and the Angel of Miracles
Ζεύς The Angel of Cycles and Solutions will help us get back on track. In the old schools this angel was known as Jupiter (Zeus in the Greek Mysteries) and was thought of as the personification of cyclic law, the Causal Power of expansion, and the angel of miracles. Price, John Randolph (2010-11-24). Angels Within Us: A Spiritual Guide to the Twenty-Two Angels That Govern Our Everyday Lives (p. 151). Random House Publishing Group. Kindle Edition. Zeus 1 Zeus For other uses, see Zeus (disambiguation). Zeus God of the sky, lightning, thunder, law, order, justice [1] The Jupiter de Smyrne, discovered in Smyrna in 1680 Abode Mount Olympus Symbol Thunderbolt, eagle, bull, and oak Consort Hera and various others Parents Cronus and Rhea Siblings Hestia, Hades, Hera, Poseidon, Demeter Children Aeacus, Ares, Athena, Apollo, Artemis, Aphrodite, Dardanus, Dionysus, Hebe, Hermes, Heracles, Helen of Troy, Hephaestus, Perseus, Minos, the Muses, the Graces [2] Roman equivalent Jupiter Zeus (Ancient Greek: Ζεύς, Zeús; Modern Greek: Δίας, Días; English pronunciation /ˈzjuːs/[3] or /ˈzuːs/) is the "Father of Gods and men" (πατὴρ ἀνδρῶν τε θεῶν τε, patḕr andrōn te theōn te)[4] who rules the Olympians of Mount Olympus as a father rules the family according to the ancient Greek religion. He is the god of sky and thunder in Greek mythology. Zeus is etymologically cognate with and, under Hellenic influence, became particularly closely identified with Roman Jupiter. Zeus is the child of Cronus and Rhea, and the youngest of his siblings. In most traditions he is married to Hera, although, at the oracle of Dodona, his consort is Dione: according to the Iliad, he is the father of Aphrodite by Dione.[5] He is known for his erotic escapades. -
Bulfinch's Mythology
Bulfinch's Mythology Thomas Bulfinch Bulfinch's Mythology Table of Contents Bulfinch's Mythology..........................................................................................................................................1 Thomas Bulfinch......................................................................................................................................1 PUBLISHERS' PREFACE......................................................................................................................3 AUTHOR'S PREFACE...........................................................................................................................4 STORIES OF GODS AND HEROES..................................................................................................................7 CHAPTER I. INTRODUCTION.............................................................................................................7 CHAPTER II. PROMETHEUS AND PANDORA...............................................................................13 CHAPTER III. APOLLO AND DAPHNEPYRAMUS AND THISBE CEPHALUS AND PROCRIS7 CHAPTER IV. JUNO AND HER RIVALS, IO AND CALLISTODIANA AND ACTAEONLATONA2 AND THE RUSTICS CHAPTER V. PHAETON.....................................................................................................................27 CHAPTER VI. MIDASBAUCIS AND PHILEMON........................................................................31 CHAPTER VII. PROSERPINEGLAUCUS AND SCYLLA............................................................34 -
Anemoi - Wikipedia, the Free Encyclopedia
זפירוס زيفيروس زپيروس Ζέφυρος - Wiktionary http://en.wiktionary.org/wiki/ Ζέφυρος Ζέφυρος Definition from Wiktionary, the free dictionary Contents 1 Ancient Greek 1.1 Etymology 1.2 Pronunciation 1.3 Proper noun 1.3.1 Inflection 1.3.2 Descendants 1.4 References Ancient Greek Etymology Probably related to ζόφος ( zóphos , “darkness, west”) Pronunciation (5th BC Attic ): IPA: /zdépʰyros/ (1st BC Egyptian ): IPA: /zɛ́pʰyros/ (4th AD Koine ): IPA: /zéɸyros/ (10th AD Byzantine ): IPA: /zéfyros/ (15th AD Constantinopolitan ): IPA: /zéfiɾos/ Proper noun Ζέφυρος • (Zéphuros) ( genitive Ζεφύρου ) m, second declension 1. Zephyrus, the west wind Inflection Second declension of Ζέφῠρος , Ζεφύρου [show ▼] Descendants Breton: Zefyros Japanese: ゼピュロス (Zepyurosu) 1 of 2 2/26/2015 6:59 PM Ζέφυρος - Wiktionary http://en.wiktionary.org/wiki/ Ζέφυρος Czech: Zefyros Latin: Zephyrus Dutch: Zephyros Lithuanian: Zefyras English: Zephyrus, Zephyr Old Armenian: զեփիւռ (zep ʿiw ṙ) French: Zéphyr Armenian: զեփյուռ (zep ʿyu ṙ) German: Zephyr Polish: Zefir Greek: Ζέφυρος (Zéfyros) Portuguese: Zéfiro Spanish: Céfiro זפירוס , זפיר :Hebrew Hungarian: Zephürosz Swedish: Zefyros Italian: Zefiro References Woodhouse’s English-Greek Dictionary page 1029 (http://artflx.uchicago.edu/cgi-bin/efts/dicos /woodhouse_test.pl?pageturn=1&pagenumber=1029) Retrieved from "http://en.wiktionary.org/w/index.php?title= Ζέφυρος &oldid=23089011" Categories: Ancient Greek lemmas Ancient Greek proper nouns Ancient Greek second declension proper nouns This page was last modified on 19 September -
Speaking to the Four Winds! God Spoke to All Kinds of Things
SPEAKING TO THE FOUR WINDS! GOD SPOKE TO ALL KINDS OF THINGS • 3 And God said, Let there be light: and there was light. • 6 And God said, Let there be a firmament in the midst of the waters, and there it was…. • 9 And God said, Let the waters under the heaven be gathered together unto one place, and let the dry land appear: and it was so. • 11 And God said, Verse 14, 20, 24 • 26 And God said, Let us make man in our image, after our likeness ,… with the power and abilities like us to speak to things! JESUS SPOKE TO ALL KINDS OF THINGS Jesus spoke to the fig tree - Mark 11:14 Mark 4:39 Then He arose and rebuked the wind, and said to the sea, “Peace, be still!” And the wind ceased and there was a great calm. He spoke to a dead body John 11:43. called Lazarus SONSHIP • Not that I should do My will, but the will of Him that has sent Me. John. 6: 38 • The Son can do nothing of Himself save whatever He sees the Father doing. John 5: 19.. • For I have not spoken from Myself, but the Father who sent Me has Himself given Me commandment what I should say and what I should speak” (Jn. 12: 49) JESUS’S PRINCIPAL OF SPEAKING TO THINGS. 22 So Jesus answered and said to them, “Have faith in God. 23 For assuredly, I say to you, whoever says to this mountain, ‘Be removed and be cast into the sea,’ and does not doubt in his heart, but believes that those things he says will be done, he will have whatever he says. -
Indian Princess Passage Ceremony
INDIAN PRINCESS PASSAGE CEREMONY (BB) = Big Brave (LP) = Little Princess Tom-Tom and Lead Runner/Torchbearer: Lead procession to Council Ring. Along the way, the tom-tom beats slowly and the Lead Runner (Torchbearer) recites the message below at each tribe’s cabin. Lead Runner (BB): Chief (dad’s Indian name) of the mighty (your tribe name) invites members of our Federation making passage in our program to join us at the council ring. When the torchbearer/leadrunner arrives at the council ring, please wait for all behind to gather up towards the council ring and then Ceremony Chief will start. Ceremony Chief (BB): Who approaches our sacred Council Ring? Lead Runner (BB): Oh Great Chief of the Sacred Council Ring, I Chief (dad’s Indian name), bring Princesses and Big Braves who have proven their worth and wish to make passage from our Federation. Ceremony Chief (LP): Enter all who are worthy. Tom-Tom beats drum slowly until all have entered and are seated in the council ring. Ceremony Chief (BB): Welcome Big Braves and Princesses to our Council Ring. Join us now as we call up the Great Spirit to be with us tonight. Medicine Man (BB): Oh Great Spirit, be with us now as these Fathers and Daughters bind themselves to be “Friends Always”. Help us to sacrifice selfishness and impatience tonight and always. Medicine Man (LP): Great Spirit, send the hovering spirit for guidance and direction. Ceremony Chief (BB): If the Great Spirit is satisfied that all within our Council Ring are worthy, we ask him to give us a sign. -
Four Classifications of Prophets
THE PROPHETIC REALM SERIES FOUR CLASSIFICATIONS OF PROPHETS Tonight we want to look at the life of a prophet. And if you have been reading the word of God consistently, you may have noticed that one of the prominent prophets in the bible is the prophet Elijah. In the New Testament John the Baptist was supposed to have the spirit of Elijah. If you examine it very carefully John the Baptist did not do the same kind of miracles that Elijah did. John the Baptist preached repentance. Yet the bible says that he has the spirit of Elijah even though he did not do all those things that Elijah and Elisha did. So as I look into all these prophets and their lives, God began to show that there are two types of prophets in Old Testament. And there are four flavors of prophet. Lets look at First Chron. 29:29 It says of the life of David who himself was a king and a prophet. Verse 29 Now the acts of king David first and last indeed they are written in the book of Samuel the seer, in the book of Nathan the prophet, and in the book of Gad the seer. Although the bible tells us in the book of First Samuel chapter 16 and in chapter 9 it tells that in those days they call a prophet a seer. And they use the word seer and prophet synonymously. We have always looked on those two words as the same. Notice here that they purposely isolate Samuel as a seer, Gad as a seer and Nathan as a prophet. -
Bulfinch's Mythology the Age of Fable by Thomas Bulfinch
1 BULFINCH'S MYTHOLOGY THE AGE OF FABLE BY THOMAS BULFINCH Table of Contents PUBLISHERS' PREFACE ........................................................................................................................... 3 AUTHOR'S PREFACE ................................................................................................................................. 4 INTRODUCTION ........................................................................................................................................ 7 ROMAN DIVINITIES ............................................................................................................................ 16 PROMETHEUS AND PANDORA ............................................................................................................ 18 APOLLO AND DAPHNE--PYRAMUS AND THISBE CEPHALUS AND PROCRIS ............................ 24 JUNO AND HER RIVALS, IO AND CALLISTO--DIANA AND ACTAEON--LATONA AND THE RUSTICS .................................................................................................................................................... 32 PHAETON .................................................................................................................................................. 41 MIDAS--BAUCIS AND PHILEMON ....................................................................................................... 48 PROSERPINE--GLAUCUS AND SCYLLA ............................................................................................. 53 PYGMALION--DRYOPE-VENUS -
1 the Ruah of Eloim
The Ruah of Eloim: A Prolegomenon to a Salesian Theology of the Word1 Alexander T. Pocetto, OSFS Presented at the Salesian Scholars Seminar Stella Niagara, NY October 2018 Nature and the Elements St. Francis de Sales (1567-1622) had a great love for nature and creation. He saw creation essentially as a manifestation of the wonder, power and love of God.2 Anyone who has ever visited Annecy with its lake and majestic mountains can readily understand the reason. It is one of the most beautiful places on the European continent. With such magnificent scenery, one can easily appreciate why the people of Annecy would feel very close to nature. They are especially conscious of the wind. The north wind is so well-known that it has a special name. Many of the people living in Annecy speak of their illnesses and discomfort as being caused by la bise, the north wind. In the south, it is the sirocco or le vent du midi which blows constantly, especially in the summer. Anyone who has been at the Avignon summer festival can readily attest to this. The Holy Spirit and the Elements Like St. Francis de Sales, the people of biblical times were very close to nature and to the elements. They attributed the awesome power in nature, especially of the elements – wind, fire, water and storms – to be the direct actions and interventions of God. If we view nature the way the people of the Old Testament did, then we might get a less elusive understanding of God, of the Holy Spirit, and of a theology of the Word.