Extracts of Both the Cytoplasmic and Outer Membranes of Es
Total Page:16
File Type:pdf, Size:1020Kb
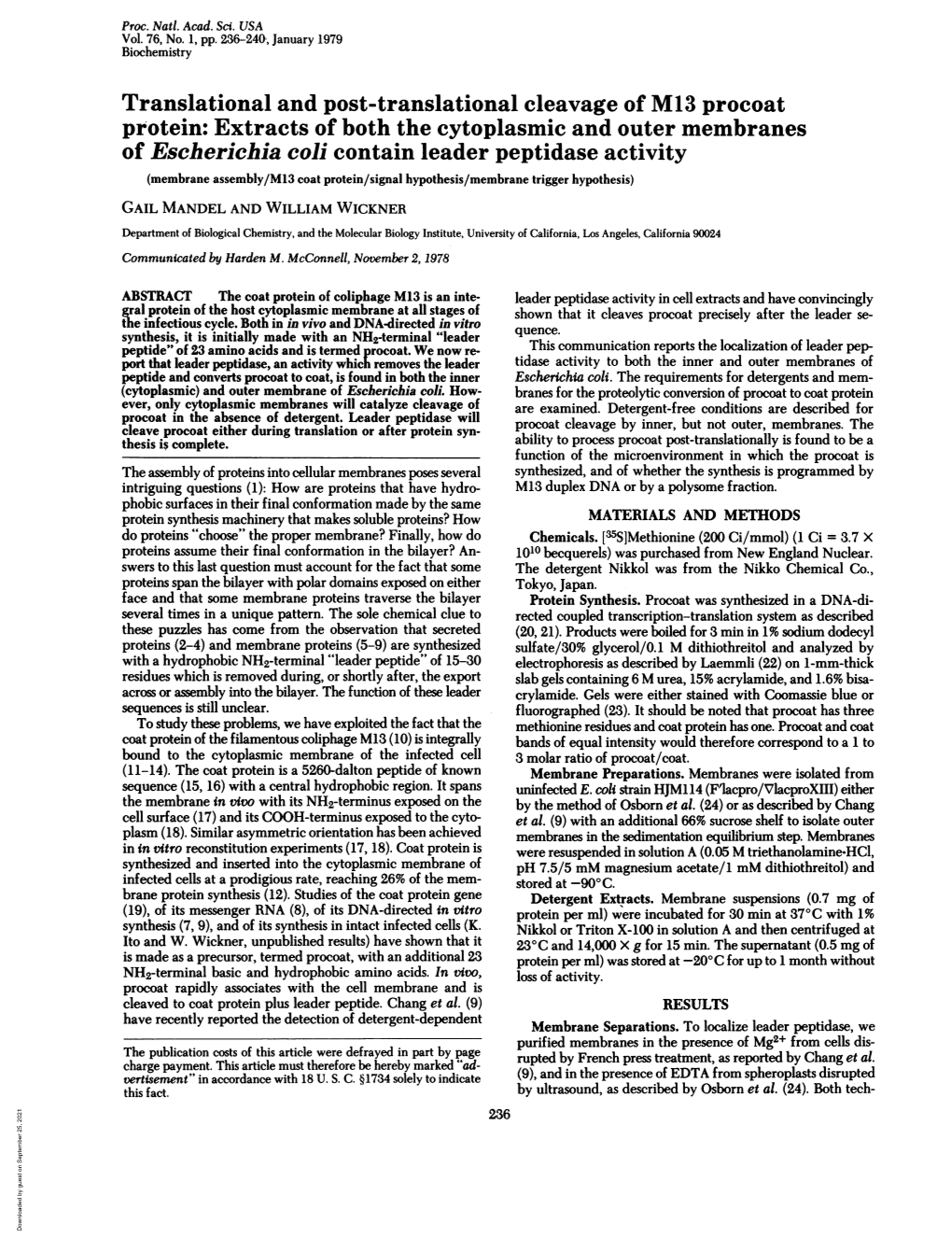
Load more
Recommended publications
-
L-Leucine Increases Translation of RPS14 and LARP1 in Erythroblasts
LETTERS TO THE EDITOR Table 1. Top 20 differentially translated known 5’TOP mRNAs in L-leucine increases translation of RPS14 and LARP1 L-leucine treated erythroblasts from del(5q) myelodysplastic syn- in erythroblasts from del(5q) myelodysplastic drome patients. syndrome patients Genes LogFC of TE in patients z score patients Deletion of the long arm of chromosome 5 [del(5q)] is RPS15 3.55 2.46 the most common cytogenetic abnormality found in the RPS27A 3.48 2.40 1 myelodysplastic syndromes (MDS). Patients with the 5q- RPS25 3.47 2.39 syndrome have macrocytic anemia and the del(5q) as the RPS20 3.43 2.35 sole karyotypic abnormality.1 Haploinsufficiency of the ribosomal protein gene RPS14, mapping to the common- RPL12 3.35 2.29 ly deleted region (CDR) on chromosome 5q,2 underlies PABPC4 3.01 2.01 3 the erythroid defect found in the 5q- syndrome, and is RPS24 2.97 1.98 associated with p53 activation,4-6 a block in the process- ing of pre-ribosomal RNA,3 and deregulation of riboso- RPS3 2.95 1.96 mal- and translation-related genes.7 Defective mRNA EEF2 2.83 1.86 translation represents a potential therapeutic target in the RPS18 2.76 1.80 5q- syndrome and other ribosomopathies, such as RPS26 2.75 1.79 Diamond-Blackfan anemia (DBA).8 Evidence suggests that the translation enhancer L- RPS5 2.69 1.74 leucine may have some efficacy in the treatment of the RPS21 2.64 1.70 8 5q- syndrome and DBA. A DBA patient treated with L- RPS9 2.54 1.62 leucine showed a marked improvement in anemia and 8 EIF3E 2.53 1.61 achieved transfusion independence. -
Evolution of Translation EF-Tu: Trna
University of Illinois at Urbana-Champaign Luthey-Schulten Group NIH Resource for Macromolecular Modeling and Bioinformatics Computational Biophysics Workshop Evolution of Translation EF-Tu: tRNA VMD Developer: John Stone MultiSeq Developers Tutorial Authors Elijah Roberts Ke Chen John Eargle John Eargle Dan Wright Zhaleh Ghaemi Jonathan Lai Zan Luthey-Schulten August 2014 A current version of this tutorial is available at http://www.scs.illinois.edu/~schulten/tutorials/ef-tu CONTENTS 2 Contents 1 Introduction 3 1.1 The Elongation Factor Tu . 3 1.2 Getting Started . 4 1.2.1 Requirements . 4 1.2.2 Copying the tutorial files . 4 1.2.3 Working directory . 4 1.2.4 Preferences . 4 1.3 Configuring BLAST for MultiSeq . 5 2 Comparative Analysis of EF-Tu 5 2.1 Finding archaeal EF1A sequences . 6 2.2 Aligning archaeal sequences and removing redundancy . 8 2.3 Finding bacteria EF-Tu sequences . 11 2.4 Performing ClustalW Multiple Sequence and Profile-Profile Align- ments . 12 2.5 Creating Multiple Sequence with MAFFT . 16 2.6 Conservation of EF-Tu among the Bacteria . 16 2.7 Finding conserved residues across the bacterial and archaeal do- mains . 20 2.8 EF-Tu Interface with the Ribosome . 21 3 Computing a Maximum Likelihood Phylogenetic Tree with RAxML 23 3.1 Load the Phylogenetic Tree into MultiSeq . 25 3.2 Reroot and Manipulate the Phylogenetic Tree . 25 4 MultiSeq TCL Scripting: Genomic Context 27 5 Appendix A 30 5.1 Building a BLAST Database . 30 6 Appendix B 31 6.1 Saving QR subset of alignments in PHYLIP and FASTA format 31 6.2 Calculating Maximum Likelihood Trees with RAxML . -
Dynamics of Translation Can Determine the Spatial Organization Of
Dynamics of translation can determine the spatial organization of membrane-bound proteins and their mRNA Elgin Korkmazhana, Hamid Teimourib,c, Neil Petermanb,c, and Erel Levineb,c,1 aHarvard College, Harvard University, Cambridge, MA 02138; bDepartment of Physics, Harvard University, Cambridge, MA 02138; and cFAS Center for Systems Biology, Harvard University, Cambridge, MA 02138 Edited by William Bialek, Princeton University, Princeton, NJ, and approved October 17, 2017 (received for review January 17, 2017) Unlike most macromolecules that are homogeneously distributed these patterns are determined by the organization of the cod- in the bacterial cell, mRNAs that encode inner-membrane proteins ing sequence, the presence of slow codons, the rate of transla- can be concentrated near the inner membrane. Cotranslational tion initiation, and the availability of auxiliary proteins required insertion of the nascent peptide into the membrane brings the for membrane targeting (referred to as the secretory machinery). translating ribosome and the mRNA close to the membrane. This By calculating the distribution of the number of proteins placed suggests that kinetic properties of translation can determine the together in the membrane, we suggest implications of mRNA spatial organization of these mRNAs and proteins, which can be localization on the organization of proteins on the membrane. modulated through posttranscriptional regulation. Here we use a We thus propose a mechanism for the formation of protein clus- simple stochastic model of translation to characterize the effect of ters in the membrane and investigate its implications on the reg- mRNA properties on the dynamics and statistics of its spatial distri- ulation of their size distribution. -
USP16 Counteracts Mono-Ubiquitination of Rps27a And
RESEARCH ARTICLE USP16 counteracts mono-ubiquitination of RPS27a and promotes maturation of the 40S ribosomal subunit Christian Montellese1†, Jasmin van den Heuvel1,2, Caroline Ashiono1, Kerstin Do¨ rner1,2, Andre´ Melnik3‡, Stefanie Jonas1§, Ivo Zemp1, Paola Picotti3, Ludovic C Gillet1, Ulrike Kutay1* 1Institute of Biochemistry, ETH Zurich, Zurich, Switzerland; 2Molecular Life Sciences Ph.D. Program, Zurich, Switzerland; 3Institute of Molecular Systems Biology, ETH Zurich, Zurich, Switzerland Abstract Establishment of translational competence represents a decisive cytoplasmic step in the biogenesis of 40S ribosomal subunits. This involves final 18S rRNA processing and release of residual biogenesis factors, including the protein kinase RIOK1. To identify novel proteins promoting the final maturation of human 40S subunits, we characterized pre-ribosomal subunits trapped on RIOK1 by mass spectrometry, and identified the deubiquitinase USP16 among the captured factors. We demonstrate that USP16 constitutes a component of late cytoplasmic pre-40S subunits that promotes the removal of ubiquitin from an internal lysine of ribosomal protein *For correspondence: RPS27a/eS31. USP16 deletion leads to late 40S subunit maturation defects, manifesting in [email protected] incomplete processing of 18S rRNA and retarded recycling of late-acting ribosome biogenesis Present address: †CSL Behring, factors, revealing an unexpected contribution of USP16 to the ultimate step of 40S synthesis. CSL Biologics Research Center, Finally, ubiquitination of RPS27a appears to depend on active translation, pointing at a potential ‡ Bern, Switzerland; MSD Merck connection between 40S maturation and protein synthesis. Sharp & Dohme AG, Lucerne, Switzerland; §Institute of Molecular Biology and Biophysics, ETH Zurich, Zurich, Switzerland Introduction Ribosomes stand at the center of translation in all kingdoms of life, catalyzing the synthesis of pro- Competing interests: The teins by reading a messenger RNA (mRNA) template. -
Archaeal Translation Initiation Revisited: the Initiation Factor 2 and Eukaryotic Initiation Factor 2B ␣--␦ Subunit Families
Proc. Natl. Acad. Sci. USA Vol. 95, pp. 3726–3730, March 1998 Evolution Archaeal translation initiation revisited: The initiation factor 2 and eukaryotic initiation factor 2B a-b-d subunit families NIKOS C. KYRPIDES* AND CARL R. WOESE Department of Microbiology, University of Illinois at Urbana-Champaign, B103 Chemical and Life Sciences, MC 110, 407 S. Goodwin, Urbana, IL 61801 Contributed by Carl R. Woese, December 31, 1997 ABSTRACT As the amount of available sequence data bacterial and eukaryotic translation initiation mechanisms, increases, it becomes apparent that our understanding of although mechanistically generally similar, were molecularly translation initiation is far from comprehensive and that unrelated and so had evolved independently. The Methano- prior conclusions concerning the origin of the process are coccus jannaschii genome (7–9), which gave us our first wrong. Contrary to earlier conclusions, key elements of trans- comprehensive look at the componentry of archaeal transla- lation initiation originated at the Universal Ancestor stage, for tion initiation, revealed that archaeal translation initiation homologous counterparts exist in all three primary taxa. showed considerable homology with eukaryotic initiation, Herein, we explore the evolutionary relationships among the which, if anything, reinforced the divide between bacterial components of bacterial initiation factor 2 (IF-2) and eukary- initiation and that seen in the other domains. We recently have otic IF-2 (eIF-2)/eIF-2B, i.e., the initiation factors involved in shown, however, that bacterial translation initiation factor 1 introducing the initiator tRNA into the translation mecha- (IF-1), contrary to previously accepted opinion, is related in nism and performing the first step in the peptide chain sequence to its eukaryotic/archaeal (functional) counterpart, elongation cycle. -
History of the Ribosome and the Origin of Translation
History of the ribosome and the origin of translation Anton S. Petrova,1, Burak Gulena, Ashlyn M. Norrisa, Nicholas A. Kovacsa, Chad R. Berniera, Kathryn A. Laniera, George E. Foxb, Stephen C. Harveyc, Roger M. Wartellc, Nicholas V. Huda, and Loren Dean Williamsa,1 aSchool of Chemistry and Biochemistry, Georgia Institute of Technology, Atlanta, GA 30332; bDepartment of Biology and Biochemistry, University of Houston, Houston, TX, 77204; and cSchool of Biology, Georgia Institute of Technology, Atlanta, GA 30332 Edited by David M. Hillis, The University of Texas at Austin, Austin, TX, and approved November 6, 2015 (received for review May 18, 2015) We present a molecular-level model for the origin and evolution of building up of the functional centers, proceeds to the establishment the translation system, using a 3D comparative method. In this model, of the common core, and continues to the development of large the ribosome evolved by accretion, recursively adding expansion metazoan rRNAs. segments, iteratively growing, subsuming, and freezing the rRNA. Incremental evolution of function is mapped out by stepwise Functions of expansion segments in the ancestral ribosome are accretion of rRNA. In the extant ribosome, specific segments of assigned by correspondence with their functions in the extant rRNA perform specific functions including peptidyl transfer, ribosome. The model explains the evolution of the large ribosomal subunit association, decoding, and energy-driven translocation subunit, the small ribosomal subunit, tRNA, and mRNA. Prokaryotic (11). The model assumes that the correlations of rRNA segments ribosomes evolved in six phases, sequentially acquiring capabilities with their functions have been reasonably maintained over the for RNA folding, catalysis, subunit association, correlated evolution, broad course of ribosomal evolution. -
The Mechanism of Eukaryotic Translation Initiation and Principles of Its Regulation
REVIEWS POST-TRANSCRIPTIONAL CONTROL The mechanism of eukaryotic translation initiation and principles of its regulation Richard J. Jackson*, Christopher U. T. Hellen‡ and Tatyana V. Pestova‡ Abstract | Protein synthesis is principally regulated at the initiation stage (rather than during elongation or termination), allowing rapid, reversible and spatial control of gene expression. Progress over recent years in determining the structures and activities of initiation factors, and in mapping their interactions in ribosomal initiation complexes, have advanced our understanding of the complex translation initiation process. These developments have provided a solid foundation for studying the regulation of translation initiation by mechanisms that include the modulation of initiation factor activity (which affects almost all scanning-dependent initiation) and through sequence-specific RNA-binding proteins and microRNAs (which affect individual mRNAs). Met Translation initiation is the process of assembly of significance is particularly high, and we include evi- Met-tRNA i The unique initiator tRNA, elongation-competent 80S ribosomes, in which the ini- dence from lower eukaryotes only when it enhances our aminoacylated with tiation codon is base-paired with the anticodon loop understanding of the mechanisms in vertebrates. methionine, that is used to Met 1 of initiator tRNA (Met-tRNA i) in the ribosomal P-site . initiate protein synthesis. Mechanism of 5′ end-dependent initiation Its anticodon is complementary It requires at least nine eukaryotic initiation factors to the AUG initiation codon; (eIFs; TABLE 1) and comprises two steps: the formation The canonical mechanism of translation initiation can be it forms a specific ternary of 48S initiation complexes with established codon– divided into several stages (FIG.1), as described below. -
Mrna Stem-Loops Can Pause the Ribosome by Hindering A-Site Trna
RESEARCH ARTICLE mRNA stem-loops can pause the ribosome by hindering A-site tRNA binding Chen Bao1†, Sarah Loerch2†, Clarence Ling1, Andrei A Korostelev3,4, Nikolaus Grigorieff2,4*, Dmitri N Ermolenko1* 1Department of Biochemistry and Biophysics at School of Medicine and Dentistry and Center for RNA Biology, University of Rochester, Rochester, United States; 2Janelia Research Campus, Howard Hughes Medical Institute, Ashburn, United States; 3Department of Biochemistry and Molecular Pharmacology, University of Massachusetts Medical School, Worcester, United States; 4RNA Therapeutics Institute, University of Massachusetts Medical School, Worcester, United States Abstract Although the elongating ribosome is an efficient helicase, certain mRNA stem-loop structures are known to impede ribosome movement along mRNA and stimulate programmed ribosome frameshifting via mechanisms that are not well understood. Using biochemical and single- molecule Fo¨ rster resonance energy transfer (smFRET) experiments, we studied how frameshift- inducing stem-loops from E. coli dnaX mRNA and the gag-pol transcript of Human Immunodeficiency Virus (HIV) perturb translation elongation. We find that upon encountering the ribosome, the stem-loops strongly inhibit A-site tRNA binding and ribosome intersubunit rotation *For correspondence: that accompanies translation elongation. Electron cryo-microscopy (cryo-EM) reveals that the HIV [email protected] (NG); stem-loop docks into the A site of the ribosome. Our results suggest that mRNA stem-loops can Dmitri_Ermolenko@urmc. transiently escape the ribosome helicase by binding to the A site. Thus, the stem-loops can rochester.edu (DNE) modulate gene expression by sterically hindering tRNA binding and inhibiting translation †These authors contributed elongation. equally to this work Competing interest: See page 22 Introduction Funding: See page 22 During translation elongation, the ribosome moves along mRNA in a codon-by-codon manner while Received: 06 February 2020 the mRNA is threaded through the mRNA channel of the small ribosomal subunit. -
Rps3/Us3 Promotes Mrna Binding at the 40S Ribosome Entry Channel and Stabilizes Preinitiation Complexes at Start Codons
Rps3/uS3 promotes mRNA binding at the 40S ribosome entry channel and stabilizes preinitiation complexes at start codons Jinsheng Donga, Colin Echeverría Aitkenb, Anil Thakura, Byung-Sik Shina, Jon R. Lorschb,1, and Alan G. Hinnebuscha,1 aLaboratory of Gene Regulation and Development, Eunice Kennedy Shriver National Institute of Child Health and Human Development, National Institutes of Health, Bethesda, MD 20892; and bLaboratory on the Mechanism and Regulation of Protein Synthesis, Eunice Kennedy Shriver National Institute of Child Health and Human Development, National Institutes of Health, Bethesda, MD 20892 Contributed by Alan G. Hinnebusch, January 24, 2017 (sent for review December 15, 2016; reviewed by Jamie H. D. Cate and Matthew S. Sachs) Met The eukaryotic 43S preinitiation complex (PIC) bearing Met-tRNAi rearrangement to PIN at both near-cognate start codons (e.g., in a ternary complex (TC) with eukaryotic initiation factor (eIF)2-GTP UUG) and cognate (AUG) codons in poor Kozak context; hence scans the mRNA leader for an AUG codon in favorable “Kozak” eIF1 must dissociate from the 40S subunit for start-codon rec- context. AUG recognition provokes rearrangement from an open ognition (Fig. 1A). Consistent with this, structural analyses of PIC conformation with TC bound in a state not fully engaged with partial PICs reveal that eIF1 and eIF1A promote rotation of the “ ” the P site ( POUT ) to a closed, arrested conformation with TC tightly 40S head relative to the body (2, 3), thought to be instrumental bound in the “P ” state. Yeast ribosomal protein Rps3/uS3 resides IN in TC binding in the POUT conformation, but that eIF1 physically in the mRNA entry channel of the 40S subunit and contacts mRNA Met clashes with Met-tRNAi in the PIN state (2, 4), and is both via conserved residues whose functional importance was unknown. -
Translation | Principles of Biology from Nature Education
contents Principles of Biology 50 Translation Translation is the process by which a cell assembles proteins from the genetic code. The Rosetta stone. To translate from one language to another, you need a set of comparative rules that act as a template. The Rosetta stone is concrete evidence of how languages were first translated in early human cultures. By displaying the same story in different languages, it served as a template for comparison, a reference for how to get from one language to another. In cells, microscopic structures called ribosomes serve as key sites that support the translation of the mRNA language into the protein language. Archiv/Photo Researchers/Science Source. Topics Covered in this Module Translating DNA into Proteins Major Objectives of this Module Describe the molecular structures involved in translation. Explain the process of translation in detail. Explain how post-translational processes prepare proteins for their functions. page 256 of 989 3 pages left in this module contents Principles of Biology 50 Translation How does a genetic code with only four nucleotides provide the information needed to generate proteins containing up to 20 different amino acids? For this process to occur, many enzymes with specific structures and function are required. Translating DNA into Proteins Translation is the process of converting the information stored in mRNA into protein (Figure 1). Proteins are made up of a series of amino acids. With the help of adapter molecules called transfer RNAs (tRNAs) the appropriate amino acid is added for each set of three adjacent nucleotides in the mRNA, called a codon (Figure 2). -
Global Analysis of LARP1 Translation Targets Reveals Tunable and Dynamic Features of 5′ TOP Motifs
Global analysis of LARP1 translation targets reveals tunable and dynamic features of 5′ TOP motifs Lucas Philippea,1, Antonia M. G. van den Elzena,1, Maegan J. Watsona, and Carson C. Thoreena,2 aDepartment of Cellular and Molecular Physiology, Yale School of Medicine, New Haven, CT 06510 Edited by Alan G. Hinnebusch, National Institutes of Health, Bethesda, MD, and approved January 29, 2020 (received for review July 25, 2019) Terminal oligopyrimidine (TOP) motifs are sequences at the 5′ ends recent findings have hinted that the RNA-binding protein La- of mRNAs that link their translation to the mTOR Complex 1 related protein 1 (LARP1) may have a central role (8–10). (mTORC1) nutrient-sensing signaling pathway. They are com- LARP1 is a large protein (150 kDa) with several RNA-binding monly regarded as discrete elements that reside on ∼100 mRNAs domains. Its central region contains a La motif (LaM) domain that mostly encode translation factors. However, the full spectrum that defines the La-related protein (LARP) superfamily, along of TOP sequences and their prevalence throughout the transcrip- with an adjacent RNA recognition motif-like (RRM-L) domain. tome remain unclear, primarily because of uncertainty over the Its C terminus encodes a domain known as the DM15 region. This mechanism that detects them. Here, we globally analyzed trans- domain is unique to LARP1 and its closely related homolog lation targets of La-related protein 1 (LARP1), an RNA-binding pro- LARP1B, and is therefore also known as the LARP1 domain (11). tein and mTORC1 effector that has been shown to repress TOP Several observations suggest that LARP1 directly represses TOP mRNA translation in a few specific cases. -
In Vitro Membrane Integration of Leader Peptidase Depends on The
pif IWHQT pif vetters RIQ @IWWUA IHWIIR sn vitro memrne integrtion of leder peptidse depends on the e mhinery nd nioni phospholipids nd n our postEtrnsltionlly Y im vn ulompenurg BD enj xFtFeF idder D enne vFtF vn lte D entoinette tF uillin D qunnr von reijne D fen de uruij¡ heprtment of fiohemistry of wemrnesD gentre for fiomemrnes nd vipid inzymologyD snstitute of fiomemrnesD treht niversityD duln VD QSVR gr trehtD he xetherlnds heprtment of fiohemistryD errhenius vortoryD tokholm niversityD EIHT WI tokholmD weden eeived S tune IWWUY revised version reeived PU tune IWWU tion IPF enioni phospholipidsD whih represent out PS7 estrt e ellEfree system sed on lyste nd memrne of the memrne lipids of iF oliD re lso involved in trnsE vesiles from isherihi oli is used to study hrteristis of lotion IQF hey stimulte the ese tivity of ee IR the memrne integrtion retion of the polytopi memrne nd re ple of interting with signl peptides ISF purE protein leder peptidse @vepAF sntegrtion into inverted inner thermoreD e¤ient in vivo trnslotion requires the presene memrne vesiles ws deteted y prtil protetion ginst externlly dded proteseF sntegrtion is most effiient when of proton motive fore @wpA IT s well s the presene of oupled to trnsltion ut n lso our postEtrnsltionlly nd two memrne proteins @eh nd epA whih my e inE depends on the tion of the proteineous e mhinery nd volved in mintining wp during trnslotion ut the ext vilility of nioni phospholipidsF vep is the first exmple of roles of whih re unknown IUF efter trnslotion the sigE memrne