Subdivision of the Neotropical Prisopodinae
Total Page:16
File Type:pdf, Size:1020Kb
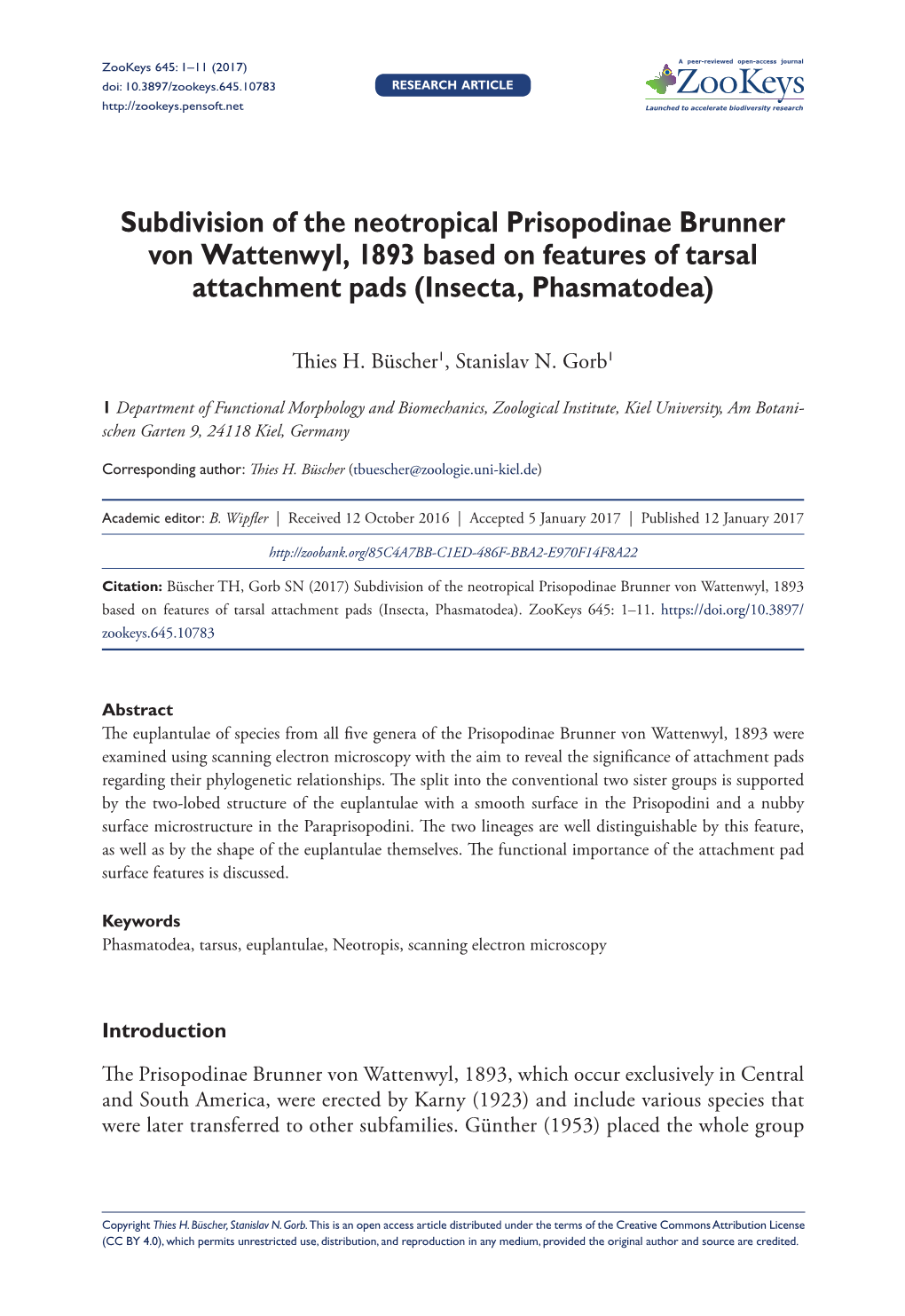
Load more
Recommended publications
-
Ecomorph Convergence in Stick Insects (Phasmatodea) with Emphasis on the Lonchodinae of Papua New Guinea
Brigham Young University BYU ScholarsArchive Theses and Dissertations 2018-07-01 Ecomorph Convergence in Stick Insects (Phasmatodea) with Emphasis on the Lonchodinae of Papua New Guinea Yelena Marlese Pacheco Brigham Young University Follow this and additional works at: https://scholarsarchive.byu.edu/etd Part of the Life Sciences Commons BYU ScholarsArchive Citation Pacheco, Yelena Marlese, "Ecomorph Convergence in Stick Insects (Phasmatodea) with Emphasis on the Lonchodinae of Papua New Guinea" (2018). Theses and Dissertations. 7444. https://scholarsarchive.byu.edu/etd/7444 This Thesis is brought to you for free and open access by BYU ScholarsArchive. It has been accepted for inclusion in Theses and Dissertations by an authorized administrator of BYU ScholarsArchive. For more information, please contact [email protected], [email protected]. Ecomorph Convergence in Stick Insects (Phasmatodea) with Emphasis on the Lonchodinae of Papua New Guinea Yelena Marlese Pacheco A thesis submitted to the faculty of Brigham Young University in partial fulfillment of the requirements for the degree of Master of Science Michael F. Whiting, Chair Sven Bradler Seth M. Bybee Steven D. Leavitt Department of Biology Brigham Young University Copyright © 2018 Yelena Marlese Pacheco All Rights Reserved ABSTRACT Ecomorph Convergence in Stick Insects (Phasmatodea) with Emphasis on the Lonchodinae of Papua New Guinea Yelena Marlese Pacheco Department of Biology, BYU Master of Science Phasmatodea exhibit a variety of cryptic ecomorphs associated with various microhabitats. Multiple ecomorphs are present in the stick insect fauna from Papua New Guinea, including the tree lobster, spiny, and long slender forms. While ecomorphs have long been recognized in phasmids, there has yet to be an attempt to objectively define and study the evolution of these ecomorphs. -
Insecta: Phasmatodea) and Their Phylogeny
insects Article Three Complete Mitochondrial Genomes of Orestes guangxiensis, Peruphasma schultei, and Phryganistria guangxiensis (Insecta: Phasmatodea) and Their Phylogeny Ke-Ke Xu 1, Qing-Ping Chen 1, Sam Pedro Galilee Ayivi 1 , Jia-Yin Guan 1, Kenneth B. Storey 2, Dan-Na Yu 1,3 and Jia-Yong Zhang 1,3,* 1 College of Chemistry and Life Science, Zhejiang Normal University, Jinhua 321004, China; [email protected] (K.-K.X.); [email protected] (Q.-P.C.); [email protected] (S.P.G.A.); [email protected] (J.-Y.G.); [email protected] (D.-N.Y.) 2 Department of Biology, Carleton University, Ottawa, ON K1S 5B6, Canada; [email protected] 3 Key Lab of Wildlife Biotechnology, Conservation and Utilization of Zhejiang Province, Zhejiang Normal University, Jinhua 321004, China * Correspondence: [email protected] or [email protected] Simple Summary: Twenty-seven complete mitochondrial genomes of Phasmatodea have been published in the NCBI. To shed light on the intra-ordinal and inter-ordinal relationships among Phas- matodea, more mitochondrial genomes of stick insects are used to explore mitogenome structures and clarify the disputes regarding the phylogenetic relationships among Phasmatodea. We sequence and annotate the first acquired complete mitochondrial genome from the family Pseudophasmati- dae (Peruphasma schultei), the first reported mitochondrial genome from the genus Phryganistria Citation: Xu, K.-K.; Chen, Q.-P.; Ayivi, of Phasmatidae (P. guangxiensis), and the complete mitochondrial genome of Orestes guangxiensis S.P.G.; Guan, J.-Y.; Storey, K.B.; Yu, belonging to the family Heteropterygidae. We analyze the gene composition and the structure D.-N.; Zhang, J.-Y. -
Could Medauroidea Extradentata (Brunner Von Wattenwyl 1907) Survive in Provence (France)? Gabriel Olive, Gilles Olive
Could Medauroidea extradentata (Brunner von Wattenwyl 1907) survive in Provence (France)? Gabriel Olive, Gilles Olive To cite this version: Gabriel Olive, Gilles Olive. Could Medauroidea extradentata (Brunner von Wattenwyl 1907) survive in Provence (France)?. Phasmid Studies, Phasmid Studies Group, 2019, 20, pp.4-14. hal-01995824 HAL Id: hal-01995824 https://hal.archives-ouvertes.fr/hal-01995824 Submitted on 27 Jan 2019 HAL is a multi-disciplinary open access L’archive ouverte pluridisciplinaire HAL, est archive for the deposit and dissemination of sci- destinée au dépôt et à la diffusion de documents entific research documents, whether they are pub- scientifiques de niveau recherche, publiés ou non, lished or not. The documents may come from émanant des établissements d’enseignement et de teaching and research institutions in France or recherche français ou étrangers, des laboratoires abroad, or from public or private research centers. publics ou privés. Phasmid Studies 20 Could Medauroidea extradentata (Brunner von Wattenwyl 1907) survive in Provence (France)? Gabriel Olive and Gilles Olive École Industrielle et Commerciale de la Ville de Namur, Laboratoire C2A, 2B, Rue Pépin, B-5000 Namur, Belgium. [email protected] Abstract Several cases of accidental introduction of insects far away from their biotope have already been re- ported. Could Medauroidea extradentata, a stick insect originally from the district of Annam, Viet- nam, survive in Provence (France)? To answer this question, two sets of experiments were performed. In the first one, specimens were put together with five plants growing only in the Mediterranean areas (bear’s breeches (Acanthus mollis), almond tree (Prunus dulcis), fig blanche d’Argenteuil variety (Ficus carica), fig violette de Solliès variety (Ficus carica), olive (Olea europaea), Aleppo pine (Pinus halepensis)) to investigate their adaptability to these diets. -
Insect Egg Size and Shape Evolve with Ecology but Not Developmental Rate Samuel H
ARTICLE https://doi.org/10.1038/s41586-019-1302-4 Insect egg size and shape evolve with ecology but not developmental rate Samuel H. Church1,4*, Seth Donoughe1,3,4, Bruno A. S. de Medeiros1 & Cassandra G. Extavour1,2* Over the course of evolution, organism size has diversified markedly. Changes in size are thought to have occurred because of developmental, morphological and/or ecological pressures. To perform phylogenetic tests of the potential effects of these pressures, here we generated a dataset of more than ten thousand descriptions of insect eggs, and combined these with genetic and life-history datasets. We show that, across eight orders of magnitude of variation in egg volume, the relationship between size and shape itself evolves, such that previously predicted global patterns of scaling do not adequately explain the diversity in egg shapes. We show that egg size is not correlated with developmental rate and that, for many insects, egg size is not correlated with adult body size. Instead, we find that the evolution of parasitoidism and aquatic oviposition help to explain the diversification in the size and shape of insect eggs. Our study suggests that where eggs are laid, rather than universal allometric constants, underlies the evolution of insect egg size and shape. Size is a fundamental factor in many biological processes. The size of an 526 families and every currently described extant hexapod order24 organism may affect interactions both with other organisms and with (Fig. 1a and Supplementary Fig. 1). We combined this dataset with the environment1,2, it scales with features of morphology and physi- backbone hexapod phylogenies25,26 that we enriched to include taxa ology3, and larger animals often have higher fitness4. -
VKM Rapportmal
VKM Report 2016: 36 Assessment of the risks to Norwegian biodiversity from the import and keeping of terrestrial arachnids and insects Opinion of the Panel on Alien Organisms and Trade in Endangered species of the Norwegian Scientific Committee for Food Safety Report from the Norwegian Scientific Committee for Food Safety (VKM) 2016: Assessment of risks to Norwegian biodiversity from the import and keeping of terrestrial arachnids and insects Opinion of the Panel on Alien Organisms and Trade in Endangered species of the Norwegian Scientific Committee for Food Safety 29.06.2016 ISBN: 978-82-8259-226-0 Norwegian Scientific Committee for Food Safety (VKM) Po 4404 Nydalen N – 0403 Oslo Norway Phone: +47 21 62 28 00 Email: [email protected] www.vkm.no www.english.vkm.no Suggested citation: VKM (2016). Assessment of risks to Norwegian biodiversity from the import and keeping of terrestrial arachnids and insects. Scientific Opinion on the Panel on Alien Organisms and Trade in Endangered species of the Norwegian Scientific Committee for Food Safety, ISBN: 978-82-8259-226-0, Oslo, Norway VKM Report 2016: 36 Assessment of risks to Norwegian biodiversity from the import and keeping of terrestrial arachnids and insects Authors preparing the draft opinion Anders Nielsen (chair), Merethe Aasmo Finne (VKM staff), Maria Asmyhr (VKM staff), Jan Ove Gjershaug, Lawrence R. Kirkendall, Vigdis Vandvik, Gaute Velle (Authors in alphabetical order after chair of the working group) Assessed and approved The opinion has been assessed and approved by Panel on Alien Organisms and Trade in Endangered Species (CITES). Members of the panel are: Vigdis Vandvik (chair), Hugo de Boer, Jan Ove Gjershaug, Kjetil Hindar, Lawrence R. -
PHASMID STUDIES Volume 20
Printed ISSN 0966-0011 Online ISSN 1750-3329 PHASMID STUDIES Volume 20. January 2019. Editors: Edward Baker & Judith Marshall Phasmid Studies 20 Bacillus atticus Brunner von Wattenwyl, 1882: A New Species for the Albanian Fauna (Phasmida: Bacillidae) Slobodan Ivković Department of Biogeography, Trier University, Universitätsring 15, 54286 Trier, Germany [email protected] Eridan Xharahi Lagja 28 Nentori, Rruga Kristo Negovani, p. 215 Vlorë, Albania [email protected] Abstract The present study represents the first report of the presence of Bacillus atticus Brunner von Wattenwyl, 1882 in Albania. Key words Distribution, Pistacia lentiscus, Vlorë, stick insects. According to PSF (2018) the stick insects (order Phasmida) are represented worldwide with 3286 valid species and in Europe with 19 species. The most common phasmid genus in Europe is Bacillus Berthold, 1827, and it is represented with six species (atticus, grandii, inermis, lynceorum, rossius and whitei), reported from central and eastern parts of the Mediterranean Basin. Bacillus species are characterized by the slightly narrowed head, smooth or granulated pronotum which is longer than wide, strongly elongated meso and metanotum, tapered subgenital plate and short, stout cerci (Harz & Kaltenbach, 1976: 15, 18; Brock, 1994: 103). Herein, we record for the first timeB. atticus Brunner von Wattenwyl, 1882 for Albania. The new record is based on a photo of a female specimen taken on 11 VIII 2014, by EH and uploaded on iN- aturalist and Facebook page “Regjistri Elektronik i Specieve Shqiptare” (Fig. 1A-C). The specimen was observed on Jal beach, Vuno village, Vlorë region, Albania (40°06’51.7”N, 19°42’04.7”E). -
Survival Meal for Medauroidea Extradentata (Brunner Von Wattenwyl 1907) (Phasmatodea: Phasmatidae) Gabriel Olive, Jean-Yves Zimmer, Gilles Olive
Mentha spicata var. spicata (L. 1753) and Raphanus sativus var. sativus (L. 1753) : survival meal for Medauroidea extradentata (Brunner von Wattenwyl 1907) (Phasmatodea: Phasmatidae) Gabriel Olive, Jean-Yves Zimmer, Gilles Olive To cite this version: Gabriel Olive, Jean-Yves Zimmer, Gilles Olive. Mentha spicata var. spicata (L. 1753) and Raphanus sativus var. sativus (L. 1753) : survival meal for Medauroidea extradentata (Brunner von Watten- wyl 1907) (Phasmatodea: Phasmatidae). Communications in Agricultural and Applied Biological Sciences, University Gent, 2016, 81 (1), pp.190-193. hal-01270314 HAL Id: hal-01270314 https://hal.archives-ouvertes.fr/hal-01270314 Submitted on 17 Feb 2016 HAL is a multi-disciplinary open access L’archive ouverte pluridisciplinaire HAL, est archive for the deposit and dissemination of sci- destinée au dépôt et à la diffusion de documents entific research documents, whether they are pub- scientifiques de niveau recherche, publiés ou non, lished or not. The documents may come from émanant des établissements d’enseignement et de teaching and research institutions in France or recherche français ou étrangers, des laboratoires abroad, or from public or private research centers. publics ou privés. 190 MENTHA SPICATA VAR. SPICATA (L. 1753) AND RAPHANUS SATIVUS VAR. SATIVUS (L. 1753): SURVIVAL MEAL FOR MEDAUROIDEA EXTRADENTATA (BRUNNER VON WATTENWYL 1907) (PHASMATODEA: PHASMATIDAE) GABRIEL OLIVE*, JEAN-YVES ZIMMER**, GILLES OLIVE* *Ecole Industrielle et Commerciale de la Ville de Namur, Laboratoire C2A, Rue Pépin, -
The Stick Insect Genus Medauroidea Zompro, 2000: Taxonomic Note and Extension to Laos and Cambodia with One New Species, M
Belgian Journal of Entomology 73: 1–19 (2018) ISSN: 2295-0214 www.srbe-kbve.be urn:lsid:zoobank.org:pub:09D65188-1D26-4A2C-8B9D-C44FA7B29F4E Belgian Journal of Entomology The stick insect genus Medauroidea Zompro, 2000: Taxonomic note and extension to Laos and Cambodia with one new species, M. romantica sp. nov. (Phasmida: Phasmatidae: Clitumninae) Joachim BRESSEEL¹ & Jérôme CONSTANT² 1,2 Royal Belgian Institute of Natural Sciences, O.D. Phylogeny and Taxonomy, Entomology, Vautier street 29, B-1000 Brussels, Belgium 1 E-mail:[email protected] (corresponding author) urn:lsid:zoobank.org:author:3C4EF358-9716-46F0-8575-26BE1EDE4349 2 E-mail: [email protected] urn:lsid:zoobank.org:author:6E6072A1-9415-4C8D-8E60-2504444DB290 Published: Brussels, July 09, 2018 Citation: BRESSEEL J. & CONSTANT J., 2018 - The stick insect genus Medauroidea Zompro, 2000: Taxonomic note and extension to Laos and Cambodia with one new species, M. romantica sp. nov. (Phasmida: Phasmatidae: Clitumninae). Belgian Journal of Entomology, 73: 1–19. ISSN: 1374-5514 (Print Edition) ISSN: 2295-0214 (Online Edition) The Belgian Journal of Entomology is published by the Royal Belgian Society of Entomology, a non-profit association established on April 9, 1855. Head office: Vautier street 29, B-1000 Brussels. The publications of the Society are partly sponsored by the University Foundation of Belgium. In compliance with Article 8.6 of the ICZN, printed versions of all papers are deposited in the following libraries: - Royal Library of Belgium, Boulevard de l’Empereur 4, B-1000 Brussels. - Library of the Royal Belgian Institute of Natural Sciences, Vautier street 29, B-1000 Brussels. -
Studies on Neotropical Phasmatodea XX: a New Genus and 16 New Species from French Guiana
Zootaxa 4814 (1): 001–136 ISSN 1175-5326 (print edition) https://www.mapress.com/j/zt/ Monograph ZOOTAXA Copyright © 2020 Magnolia Press ISSN 1175-5334 (online edition) https://doi.org/10.11646/zootaxa.4814.1.1 http://zoobank.org/urn:lsid:zoobank.org:pub:15CFB5E4-B48B-4DD9-A49E-D52BBC9ACC34 ZOOTAXA 4814 Studies on neotropical Phasmatodea XX: A new genus and 16 new species from French Guiana OSKAR V. CONLE1, FRANK H. HENNEMANN2, YANNICK BELLANGER3, PHILIPPE LELONG4, TONI JOURDAN5 & PABLO VALERO6 1Am Freischütz 16, 47058 Duisburg, Germany. �[email protected]; https://orcid.org/0000-0001-5609-5107; Website: www.phasmatodea.com 2Tannenwaldallee 53, 61348 Bad Homburg, Germany. �[email protected]; https://orcid.org/0000-0002-6547-4704; Website: www.phasmatodea.com 3La Ville-Jouy, 22250 Trédias, France. �[email protected]; https://orcid.org/0000-0003-1870-8704 4La Ferradou n°3, 31570 Sainte-Foy-d`Aigrefeuille, France. �[email protected]; https://orcid.org/0000-0002-9073-4512 595 chemin des Chevêches, 74150 Vallières, France. �[email protected]; https://orcid.org/0000-0001-6576-4472 6Aachrain 1, 87534 Oberstaufen, Germany. �[email protected]; https://orcid.org/0000-0002-5471-3458; Website: www.phasmatodea.com Magnolia Press Auckland, New Zealand Accepted by B. Mantovani: 25 May 2020; published: 14 Jul. 2020 OSKAR V. CONLE, FRANK H. HENNEMANN, YANNICK BELLANGER , PHILIPPE LELONG, TONI JOURDAN & PABLO VALERO Studies on neotropical Phasmatodea XX: A new genus and 16 new species from French Guiana (Zootaxa 4814) 136 pp.; 30 cm. 14 Jul. 2020 ISBN 978-1-77670-989-2 (paperback) ISBN 978-1-77670-990-8 (Online edition) FIRST PUBLISHED IN 2020 BY Magnolia Press P.O. -
Zootaxa, Revision of Oriental Phasmatodea
ZOOTAXA 1906 Revision of Oriental Phasmatodea: The tribe Pharnaciini Günther, 1953, including the description of the world's longest insect, and a survey of the family Phasmatidae Gray, 1835 with keys to the subfamilies and tribes (Phasmatodea: "Anareolatae": Phasmatidae) FRANK H. HENNEMANN & OSKAR V. CONLE Magnolia Press Auckland, New Zealand Frank H. Hennemann & Oskar V. Conle Revision of Oriental Phasmatodea: The tribe Pharnaciini Günther, 1953, including the description of the world's longest insect, and a survey of the family Phasmatidae Gray, 1835 with keys to the subfami- lies and tribes (Phasmatodea: "Anareolatae": Phasmatidae) (Zootaxa 1906) 316 pp.; 30 cm. 15 Oct. 2008 ISBN 978-1-86977-271-0 (paperback) ISBN 978-1-86977-272-7 (Online edition) FIRST PUBLISHED IN 2008 BY Magnolia Press P.O. Box 41-383 Auckland 1346 New Zealand e-mail: [email protected] http://www.mapress.com/zootaxa/ © 2008 Magnolia Press All rights reserved. No part of this publication may be reproduced, stored, transmitted or disseminated, in any form, or by any means, without prior written permission from the publisher, to whom all requests to reproduce copyright material should be directed in writing. This authorization does not extend to any other kind of copying, by any means, in any form, and for any purpose other than private research use. ISSN 1175-5326 (Print edition) ISSN 1175-5334 (Online edition) 2 · Zootaxa 1906 © 2008 Magnolia Press HENNEMANN & CONLE Zootaxa 1906: 1–316 (2008) ISSN 1175-5326 (print edition) www.mapress.com/zootaxa/ ZOOTAXA Copyright © 2008 · Magnolia Press ISSN 1175-5334 (online edition) Revision of Oriental Phasmatodea: The tribe Pharnaciini Günther, 1953, including the description of the world’s longest insect, and a survey of the family Phasmatidae Gray, 1835 with keys to the subfamilies and tribes* (Phasmatodea: “Anareolatae”: Phasmatidae) FRANK H. -
Evolutionary Morphology of the Antennal Heart in Stick and Leaf Insects (Phasmatodea) and Webspinners (Embioptera) (Insecta: Eukinolabia)
Zoomorphology https://doi.org/10.1007/s00435-021-00526-4 ORIGINAL PAPER Evolutionary morphology of the antennal heart in stick and leaf insects (Phasmatodea) and webspinners (Embioptera) (Insecta: Eukinolabia) Benjamin Wipfer1 · Sven Bradler2 · Sebastian Büsse3 · Jörg Hammel4 · Bernd R. Müller5 · Günther Pass6 Received: 28 January 2021 / Revised: 15 April 2021 / Accepted: 27 April 2021 © The Author(s) 2021 Abstract The morphology of the antennal hearts in the head of Phasmatodea and Embioptera was investigated with particular refer- ence to phylogenetically relevant key taxa. The antennal circulatory organs of all examined species have the same basic construction: they consist of antennal vessels that are connected to ampullae located in the head near the antenna base. The ampullae are pulsatile due to associated muscles, but the points of attachment difer between the species studied. All examined Phasmatodea species have a Musculus (M.) interampullaris which extends between the two ampullae plus a M. ampulloaorticus that runs from the ampullae to the anterior end of the aorta; upon contraction, all these muscles dilate the lumina of both ampullae at the same time. In Embioptera, only the australembiid Metoligotoma has an M. interampullaris. All other studied webspinners instead have a M. ampullofrontalis which extends between the ampullae and the frontal region of the head capsule; these species do not have M. ampulloaorticus. Outgroup comparison indicates that an antennal heart with a M. interampullaris is the plesiomorphic character state among Embioptera and the likely ground pattern of the taxon Eukinolabia. Antennal hearts with a M. ampullofrontalis represent a derived condition that occurs among insects only in some embiopterans. -
Evolution of Flight Morphology in Stick Insects
1 2 A tale of winglets: evolution of flight morphology in stick insects 3 4 Yu Zeng1,2,†, Conner O’Malley1, Sonal Singhal1,3, Faszly Rahim4,5, 5 Sehoon Park1, Xin Chen6,7, Robert Dudley1,8 6 7 1Department of Integrative Biology, University of California, Berkeley, CA 92870, 8 USA 9 2Schmid College of Science and Technology, Chapman University, Orange, CA 10 92866, USA 11 3 Department of Biology, CSU Dominguez Hills, Carson, CA 90747 USA 12 4Islamic Science Institute (ISI), Universiti Sains Islam Malaysia, 71800 Bandar Baru 13 Nilai, Negeri Sembilan, Malaysia 14 5Centre for Insect Systematics (CIS), Universiti Kebangsaan Malaysia, 43600 15 Bangi, Selangor, Malaysia 16 6Department of Biology, The College of Staten Island, The City University of New 17 York, NY 10314, USA 18 7Department of Biology, The Graduate School and University Center, The City 19 University of New York, NY 10016, USA 20 8Smithsonian Tropical Research Institute, Balboa, 21 Republic of Panama 22 23 †Corresponding author: [email protected] 24 25 1 26 27 Abstract 28 29 The evolutionary transition between winglessness and a full-winged morphology requires 30 selective advantage for intermediate forms. Conversely, repeated secondary wing 31 reductions among the pterygotes indicates relaxation of such selection. However, 32 evolutionary trajectories of such transitions are not well characterized. The stick insects 33 (Phasmatodea) exhibit diverse wing sizes at both interspecific and intersexual levels, and 34 thus provide a system for examining how selection on flight capability, along with other 35 selective forces, drives the evolution of flight-related morphology. Here, we examine 36 variation in relevant morphology for stick insects using data from 1100+ individuals 37 representing 765 species.