Iberian-Appalachian Connection Is the Missing Link Between Gondwana and Laurasia That Confrms a Wegenerian Pangaea Confguration Pedro Correia 1* & J
Total Page:16
File Type:pdf, Size:1020Kb
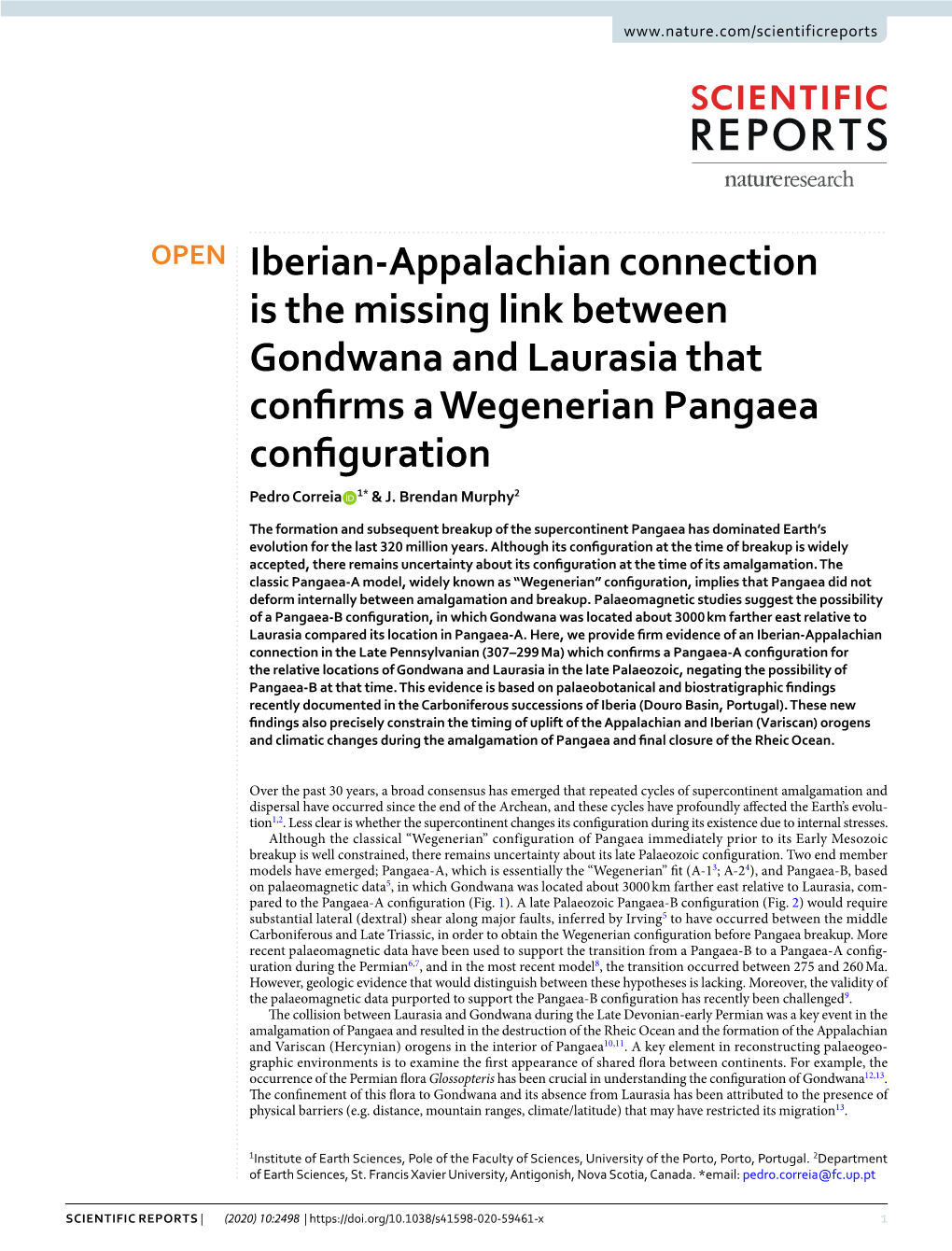
Load more
Recommended publications
-
Prepared in Cooperation with the Lllinois State Museum, Springfield
Prepared in cooperation with the lllinois State Museum, Springfield Richard 1. Leary' and Hermann W. Pfefferkorn2 ABSTRACT The Spencer Farm Flora is a compression-impression flora of early Pennsylvanian age (Namurian B, or possibly Namurian C) from Brown County, west-central Illinois. The plant fossils occur in argillaceous siltstones and sand- stones of the Caseyville Formation that were deposited in a ravine eroded in Mississippian carbonate rocks. The plant-bearing beds are the oldest deposits of Pennsylva- nian age yet discovered in Illinois. They were formed be- fore extensive Pennsylvanian coal swamps developed. The flora consists of 29 species and a few prob- lematical forms. It represents an unusual biofacies, in which the generally rare genera Megalopteris, Lesleya, Palaeopteridium, and Lacoea are quite common. Noegger- athiales, which are seldom present in roof-shale floras, make up over 20 percent of the specimens. The Spencer Farm Flora is an extrabasinal (= "upland1') flora that was grow- ing on the calcareous soils in the vicinity of the ravine in which they were deposited. It is suggested here that the Noeggerathiales may belong to the Progymnosperms and that Noeggerathialian cones might be derived from Archaeopteris-like fructifica- tions. The cone genus Lacoea is intermediate between Noeggerathiostrobus and Discini tes in its morphology. Two new species, Lesleya cheimarosa and Rhodeop- teridi urn phillipsii , are described, and Gulpenia limbur- gensis is reported from North America for the first time. INTRODUCTION The Spencer Farm Flora (table 1) differs from other Pennsylvanian floras of the Illinois Basin. Many genera and species in the Spencer Farm Flora either have not been found elsewhere in the basin or are very l~uratorof Geology, Illinois State Museum, Springfield. -
Assembly, Configuration, and Break-Up History of Rodinia
Author's personal copy Available online at www.sciencedirect.com Precambrian Research 160 (2008) 179–210 Assembly, configuration, and break-up history of Rodinia: A synthesis Z.X. Li a,g,∗, S.V. Bogdanova b, A.S. Collins c, A. Davidson d, B. De Waele a, R.E. Ernst e,f, I.C.W. Fitzsimons g, R.A. Fuck h, D.P. Gladkochub i, J. Jacobs j, K.E. Karlstrom k, S. Lu l, L.M. Natapov m, V. Pease n, S.A. Pisarevsky a, K. Thrane o, V. Vernikovsky p a Tectonics Special Research Centre, School of Earth and Geographical Sciences, The University of Western Australia, Crawley, WA 6009, Australia b Department of Geology, Lund University, Solvegatan 12, 223 62 Lund, Sweden c Continental Evolution Research Group, School of Earth and Environmental Sciences, University of Adelaide, Adelaide, SA 5005, Australia d Geological Survey of Canada (retired), 601 Booth Street, Ottawa, Canada K1A 0E8 e Ernst Geosciences, 43 Margrave Avenue, Ottawa, Canada K1T 3Y2 f Department of Earth Sciences, Carleton U., Ottawa, Canada K1S 5B6 g Tectonics Special Research Centre, Department of Applied Geology, Curtin University of Technology, GPO Box U1987, Perth, WA 6845, Australia h Universidade de Bras´ılia, 70910-000 Bras´ılia, Brazil i Institute of the Earth’s Crust SB RAS, Lermontova Street, 128, 664033 Irkutsk, Russia j Department of Earth Science, University of Bergen, Allegaten 41, N-5007 Bergen, Norway k Department of Earth and Planetary Sciences, Northrop Hall University of New Mexico, Albuquerque, NM 87131, USA l Tianjin Institute of Geology and Mineral Resources, CGS, No. -
The North-Subducting Rheic Ocean During the Devonian: Consequences for the Rhenohercynian Ore Sites
Published in "International Journal of Earth Sciences 106(7): 2279–2296, 2017" which should be cited to refer to this work. The north-subducting Rheic Ocean during the Devonian: consequences for the Rhenohercynian ore sites Jürgen F. von Raumer1 · Heinz-Dieter Nesbor2 · Gérard M. Stampfli3 Abstract Base metal mining in the Rhenohercynian Zone activated Early Devonian growth faults. Hydrothermal brines has a long history. Middle-Upper Devonian to Lower Car- equilibrated with the basement and overlying Middle-Upper boniferous sediment-hosted massive sulfide deposits Devonian detrital deposits forming the SHMS deposits in the (SHMS), volcanic-hosted massive sulfide deposits (VHMS) southern part of the Pyrite Belt, in the Rhenish Massif and and Lahn-Dill-type iron, and base metal ores occur at sev- in the Harz areas. Volcanic-hosted massive sulfide deposits eral sites in the Rhenohercynian Zone that stretches from the (VHMS) formed in the more eastern localities of the Rheno- South Portuguese Zone, through the Lizard area, the Rhen- hercynian domain. In contrast, since the Tournaisian period ish Massif and the Harz Mountain to the Moravo-Silesian of ore formation, dominant pull-apart triggered magmatic Zone of SW Bohemia. During Devonian to Early Carbonif- emplacement of acidic rocks, and their metasomatic replace- erous times, the Rhenohercynian Zone is seen as an evolv- ment in the apical zones of felsic domes and sediments in ing rift system developed on subsiding shelf areas of the the northern part of the Iberian Pyrite belt, thus changing the Old Red continent. A reappraisal of the geotectonic setting general conditions of ore precipitation. -
Structural and Tectonic Evolution of the Acatlán Complex, Southern Mexico
TECTONICS, VOL. 28, TC4008, doi:10.1029/2007TC002159, 2009 Click Here for Full Article Structural and tectonic evolution of the Acatla´n Complex, southern Mexico: Its role in the collisional history of Laurentia and Gondwana Ricardo Vega-Granillo,1 Thierry Calmus,2 Diana Meza-Figueroa,1 Joaquı´n Ruiz,3 Oscar Talavera-Mendoza,4 and Margarita Lo´pez-Martı´nez5 Received 24 May 2007; revised 22 January 2009; accepted 10 March 2009; published 25 July 2009. [1] Correlation of deformational phases and thermal opening. Citation: Vega-Granillo, R., T. Calmus, D. Meza- events in the Acatla´n Complex permits definition of Figueroa, J. Ruiz, O. Talavera-Mendoza, and M. Lo´pez-Martı´nez nine major tectonic events. Seven events are related to (2009), Structural and tectonic evolution of the Acatla´n Complex, the evolution of the Iapetus and Rheic oceans. By the southern Mexico: Its role in the collisional history of Laurentia Early Ordovician, the Xayacatla´n suite of Laurentian and Gondwana, Tectonics, 28, TC4008, doi:10.1029/ affinity was metamorphosed to eclogite facies and 2007TC002159. exhumed before colliding with the El Rodeo suite. From the Late Ordovician to Silurian, the Upper 1. Introduction Ordovician Ixcamilpa suite with peri-Gondwanan affinity was metamorphosed to blueschist facies, [2] The Acatla´n Complex basement of the Mixteco exhumed, and subsequently was overthrust by the terrane contains the largest exposure of eclogites, high-P Xayacatla´n–El Rodeo block. Part of the Esperanza garnet amphibolites, blueschists and eclogitized granitoids of Paleozoic age in Mexico. In a strict sense, the Mixteco suite is an Early Silurian intra-Iapetian continental arc terrane must be considered a superterrane composed of that collided with and subducted beneath continental thrust sheets containing Mesoproterozoic to Paleozoic pet- crust, generating a Silurian eclogitic event. -
Proterozoic East Gondwana: Supercontinent Assembly and Breakup Geological Society Special Publications Society Book Editors R
Proterozoic East Gondwana: Supercontinent Assembly and Breakup Geological Society Special Publications Society Book Editors R. J. PANKHURST (CHIEF EDITOR) P. DOYLE E J. GREGORY J. S. GRIFFITHS A. J. HARTLEY R. E. HOLDSWORTH A. C. MORTON N. S. ROBINS M. S. STOKER J. P. TURNER Special Publication reviewing procedures The Society makes every effort to ensure that the scientific and production quality of its books matches that of its journals. Since 1997, all book proposals have been refereed by specialist reviewers as well as by the Society's Books Editorial Committee. If the referees identify weaknesses in the proposal, these must be addressed before the proposal is accepted. Once the book is accepted, the Society has a team of Book Editors (listed above) who ensure that the volume editors follow strict guidelines on refereeing and quality control. We insist that individual papers can only be accepted after satis- factory review by two independent referees. The questions on the review forms are similar to those for Journal of the Geological Society. The referees' forms and comments must be available to the Society's Book Editors on request. Although many of the books result from meetings, the editors are expected to commission papers that were not pre- sented at the meeting to ensure that the book provides a balanced coverage of the subject. Being accepted for presentation at the meeting does not guarantee inclusion in the book. Geological Society Special Publications are included in the ISI Science Citation Index, but they do not have an impact factor, the latter being applicable only to journals. -
Heterospory: the Most Iterative Key Innovation in the Evolutionary History of the Plant Kingdom
Biol. Rej\ (1994). 69, l>p. 345-417 345 Printeii in GrenI Britain HETEROSPORY: THE MOST ITERATIVE KEY INNOVATION IN THE EVOLUTIONARY HISTORY OF THE PLANT KINGDOM BY RICHARD M. BATEMAN' AND WILLIAM A. DiMlCHELE' ' Departments of Earth and Plant Sciences, Oxford University, Parks Road, Oxford OXi 3P/?, U.K. {Present addresses: Royal Botanic Garden Edinburiih, Inverleith Rojv, Edinburgh, EIIT, SLR ; Department of Geology, Royal Museum of Scotland, Chambers Street, Edinburgh EHi ijfF) '" Department of Paleohiology, National Museum of Natural History, Smithsonian Institution, Washington, DC^zo^bo, U.S.A. CONTENTS I. Introduction: the nature of hf^terospon' ......... 345 U. Generalized life history of a homosporous polysporangiophyle: the basis for evolutionary excursions into hetcrospory ............ 348 III, Detection of hcterospory in fossils. .......... 352 (1) The need to extrapolate from sporophyte to gametophyte ..... 352 (2) Spatial criteria and the physiological control of heterospory ..... 351; IV. Iterative evolution of heterospory ........... ^dj V. Inter-cladc comparison of levels of heterospory 374 (1) Zosterophyllopsida 374 (2) Lycopsida 374 (3) Sphenopsida . 377 (4) PtiTopsida 378 (5) f^rogymnospermopsida ............ 380 (6) Gymnospermopsida (including Angiospermales) . 384 (7) Summary: patterns of character acquisition ....... 386 VI. Physiological control of hetcrosporic phenomena ........ 390 VII. How the sporophyte progressively gained control over the gametophyte: a 'just-so' story 391 (1) Introduction: evolutionary antagonism between sporophyte and gametophyte 391 (2) Homosporous systems ............ 394 (3) Heterosporous systems ............ 39(1 (4) Total sporophytic control: seed habit 401 VIII. Summary .... ... 404 IX. .•Acknowledgements 407 X. References 407 I. I.NIRODUCTION: THE NATURE OF HETEROSPORY 'Heterospory' sensu lato has long been one of the most popular re\ie\v topics in organismal botany. -
The Geologic Time Scale Is the Eon
Exploring Geologic Time Poster Illustrated Teacher's Guide #35-1145 Paper #35-1146 Laminated Background Geologic Time Scale Basics The history of the Earth covers a vast expanse of time, so scientists divide it into smaller sections that are associ- ated with particular events that have occurred in the past.The approximate time range of each time span is shown on the poster.The largest time span of the geologic time scale is the eon. It is an indefinitely long period of time that contains at least two eras. Geologic time is divided into two eons.The more ancient eon is called the Precambrian, and the more recent is the Phanerozoic. Each eon is subdivided into smaller spans called eras.The Precambrian eon is divided from most ancient into the Hadean era, Archean era, and Proterozoic era. See Figure 1. Precambrian Eon Proterozoic Era 2500 - 550 million years ago Archaean Era 3800 - 2500 million years ago Hadean Era 4600 - 3800 million years ago Figure 1. Eras of the Precambrian Eon Single-celled and simple multicelled organisms first developed during the Precambrian eon. There are many fos- sils from this time because the sea-dwelling creatures were trapped in sediments and preserved. The Phanerozoic eon is subdivided into three eras – the Paleozoic era, Mesozoic era, and Cenozoic era. An era is often divided into several smaller time spans called periods. For example, the Paleozoic era is divided into the Cambrian, Ordovician, Silurian, Devonian, Carboniferous,and Permian periods. Paleozoic Era Permian Period 300 - 250 million years ago Carboniferous Period 350 - 300 million years ago Devonian Period 400 - 350 million years ago Silurian Period 450 - 400 million years ago Ordovician Period 500 - 450 million years ago Cambrian Period 550 - 500 million years ago Figure 2. -
Locating South China in Rodinia and Gondwana: a Fragment of Greater India Lithosphere?
Locating South China in Rodinia and Gondwana: A fragment of greater India lithosphere? Peter A. Cawood1, 2, Yuejun Wang3, Yajun Xu4, and Guochun Zhao5 1Department of Earth Sciences, University of St Andrews, North Street, St Andrews KY16 9AL, UK 2Centre for Exploration Targeting, School of Earth and Environment, University of Western Australia, 35 Stirling Highway, Crawley, WA 6009, Australia 3State Key Laboratory of Isotope Geochemistry, Guangzhou Institute of Geochemistry, Chinese Academy of Sciences, Guangzhou 510640, China 4State Key Laboratory of Biogeology and Environmental Geology, Faculty of Earth Sciences, China University of Geosciences, Wuhan 430074, China 5Department of Earth Sciences, University of Hong Kong, Pokfulam Road, Hong Kong, China ABSTRACT metamorphosed Neoproterozoic strata and From the formation of Rodinia at the end of the Mesoproterozoic to the commencement unmetamorphosed Sinian cover (Fig. 1; Zhao of Pangea breakup at the end of the Paleozoic, the South China craton fi rst formed and then and Cawood, 2012). The Cathaysia block is com- occupied a position adjacent to Western Australia and northern India. Early Neoproterozoic posed predominantly of Neoproterozoic meta- suprasubduction zone magmatic arc-backarc assemblages in the craton range in age from ca. morphic rocks, with minor Paleoproterozoic and 1000 Ma to 820 Ma and display a sequential northwest decrease in age. These relations sug- Mesoproterozoic lithologies. Archean basement gest formation and closure of arc systems through southeast-directed subduction, resulting is poorly exposed and largely inferred from the in progressive northwestward accretion onto the periphery of an already assembled Rodinia. presence of minor inherited and/or xenocrys- Siliciclastic units within an early Paleozoic succession that transgresses across the craton were tic zircons in younger rocks (Fig. -
Assembling Laurentia—Integrated Theme Sessions on Tectonic Turning Points
Assembling Laurentia—Integrated Theme Sessions on Tectonic Turning Points Michael L. Williams, Dept. of Geosciences, University of Massachusetts, Amherst, Massachusetts 01003, USA, [email protected]; Dawn A. Kellett, Geological Survey of Canada–Atlantic Division, Natural Resources Canada/Government of Canada, 1 Challenger Drive, Dartmouth, Nova Scotia B2Y 4A2, Canada, [email protected]; Basil Tikoff*, Dept. of Geoscience, University of Wisconsin– Madison, 1215 W. Dayton Street, Madison, Wisconsin 53706, USA, [email protected]; Steven J. Whitmeyer, Dept. of Geology and Environmental Science, James Madison University, 801 Carrier Drive, MSC 6903, Harrisonburg, Virginia 22807, USA, [email protected] The North American continent records the headlined by a Pardee Symposium, which and the broader implications of, these changes, evolution of tectonic processes and tectonic will provide an overview of the tectonic evo- and to widen the scope of investigation environments from the earliest Archean to lution of Laurentia and an introduction to the beyond a particular boundary or regional geo- modern times. The continent hosts a rich concept of key “Turning Points.” Seven logical event to the scale of Laurentia itself. Archean (and possibly Hadean) record, at related topical sessions, under the general The time slices for the topical sessions are as least three great Proterozoic orogenic belts, heading “Assembling Laurentia,” will span follows (with brief explanations from each and a wide range of Phanerozoic tectonic, the GSA meeting. -
The East Asian Jigsaw Puzzle Pangaea at Risk? from Neville Haile
192 Nature Vol. 293 17 September 1981 ment with the known seismic refraction (Business and Technological Systems, Inc.) pretation of long-wavelength crustal fields and heat flow data. His group believes that magnetization model for the United States, in terms of a geological/geophysical the region is the site of a late Precambrian derived from Magsat scalar data, had much model, promises to contribute significantly rift which was reactivated in the Mesozoic better resolution than that using POGO to our understanding of the Earth's crust. to form an aulacogen and its model data. In the older disciplines of main-field involves thinning of the upper crust and an Although preliminary, the results dis modelling and studies of external fields, increase in density in the lower crust. The cussed at the meeting indicate that sep there are significant new developments in magnetic low is accounted for by either an aration of the measured field into its core, both analytical techniques and in our isotherm upwarp or, less likely, a litho crustal and external 'components' is being understanding of the physics of the field logical variation in the crust. Mayhew's achieved. The newest discipline, inter- sources. 0 The East Asian jigsaw puzzle Pangaea at risk? from Neville Haile UNTIL fairly recently, reconstructions of the world palaeogeography followed Wegener in showing Eurasia, excluding the Indian subcontinent, as a single block, with the Malay Peninsula and part or all of the Indonesian Archipelago depending from it and looking rather vulnerablel ,2. Other world palaeogeo graphical maps simply omit South-east Asia and most of China (see the figure)3,4. -
Figure 3A. Major Geologic Formations in West Virginia. Allegheney And
82° 81° 80° 79° 78° EXPLANATION West Virginia county boundaries A West Virginia Geology by map unit Quaternary Modern Reservoirs Qal Alluvium Permian or Pennsylvanian Period LTP d Dunkard Group LTP c Conemaugh Group LTP m Monongahela Group 0 25 50 MILES LTP a Allegheny Formation PENNSYLVANIA LTP pv Pottsville Group 0 25 50 KILOMETERS LTP k Kanawha Formation 40° LTP nr New River Formation LTP p Pocahontas Formation Mississippian Period Mmc Mauch Chunk Group Mbp Bluestone and Princeton Formations Ce Obrr Omc Mh Hinton Formation Obps Dmn Bluefield Formation Dbh Otbr Mbf MARYLAND LTP pv Osp Mg Greenbrier Group Smc Axis of Obs Mmp Maccrady and Pocono, undivided Burning Springs LTP a Mmc St Ce Mmcc Maccrady Formation anticline LTP d Om Dh Cwy Mp Pocono Group Qal Dhs Ch Devonian Period Mp Dohl LTP c Dmu Middle and Upper Devonian, undivided Obps Cw Dhs Hampshire Formation LTP m Dmn OHIO Ct Dch Chemung Group Omc Obs Dch Dbh Dbh Brailler and Harrell, undivided Stw Cwy LTP pv Ca Db Brallier Formation Obrr Cc 39° CPCc Dh Harrell Shale St Dmb Millboro Shale Mmc Dhs Dmt Mahantango Formation Do LTP d Ojo Dm Marcellus Formation Dmn Onondaga Group Om Lower Devonian, undivided LTP k Dhl Dohl Do Oriskany Sandstone Dmt Ot Dhl Helderberg Group LTP m VIRGINIA Qal Obr Silurian Period Dch Smc Om Stw Tonoloway, Wills Creek, and Williamsport Formations LTP c Dmb Sct Lower Silurian, undivided LTP a Smc McKenzie Formation and Clinton Group Dhl Stw Ojo Mbf Db St Tuscarora Sandstone Ordovician Period Ojo Juniata and Oswego Formations Dohl Mg Om Martinsburg Formation LTP nr Otbr Ordovician--Trenton and Black River, undivided 38° Mmcc Ot Trenton Group LTP k WEST VIRGINIA Obr Black River Group Omc Ordovician, middle calcareous units Mp Db Osp St. -
Destruction of the North China Craton
See discussions, stats, and author profiles for this publication at: https://www.researchgate.net/publication/257684968 Destruction of the North China Craton Article in Science China Earth Science · October 2012 Impact Factor: 1.49 · DOI: 10.1007/s11430-012-4516-y CITATIONS READS 69 65 6 authors, including: Rixiang Zhu Yi-Gang Xu Chinese Academy of Sciences Chinese Academy of Sciences 264 PUBLICATIONS 8,148 CITATIONS 209 PUBLICATIONS 7,351 CITATIONS SEE PROFILE SEE PROFILE Tianyu Zheng Chinese Academy of Sciences 62 PUBLICATIONS 1,589 CITATIONS SEE PROFILE All in-text references underlined in blue are linked to publications on ResearchGate, Available from: Yi-Gang Xu letting you access and read them immediately. Retrieved on: 26 May 2016 SCIENCE CHINA Earth Sciences Progress of Projects Supported by NSFC October 2012 Vol.55 No.10: 1565–1587 • REVIEW • doi: 10.1007/s11430-012-4516-y Destruction of the North China Craton ZHU RiXiang1*, XU YiGang2, ZHU Guang3, ZHANG HongFu1, XIA QunKe4 & ZHENG TianYu1 1 State Key Laboratory of Lithospheric Evolution, Institute of Geology and Geophysics, Chinese Academy of Sciences, Beijing 100029, China; 2 State Key Laboratory of Isotope Geochemistry, Guangzhou Institute of Geochemistry, Chinese Academy of Sciences, Guangzhou 510640, China; 3 School of Resource and Environmental Engineering, Hefei University of Technology, Hefei 230009, China; 4 School of Earth and Space Sciences, University of Science and Technology of China, Hefei 230026, China Received March 27, 2012; accepted June 18, 2012 A National Science Foundation of China (NSFC) major research project, Destruction of the North China Craton (NCC), has been carried out in the past few years by Chinese scientists through an in-depth and systematic observations, experiments and theoretical analyses, with an emphasis on the spatio-temporal distribution of the NCC destruction, the structure of deep earth and shallow geological records of the craton evolution, the mechanism and dynamics of the craton destruction.