Perceiving Opponent Hues in Color Induction Displays
Total Page:16
File Type:pdf, Size:1020Kb
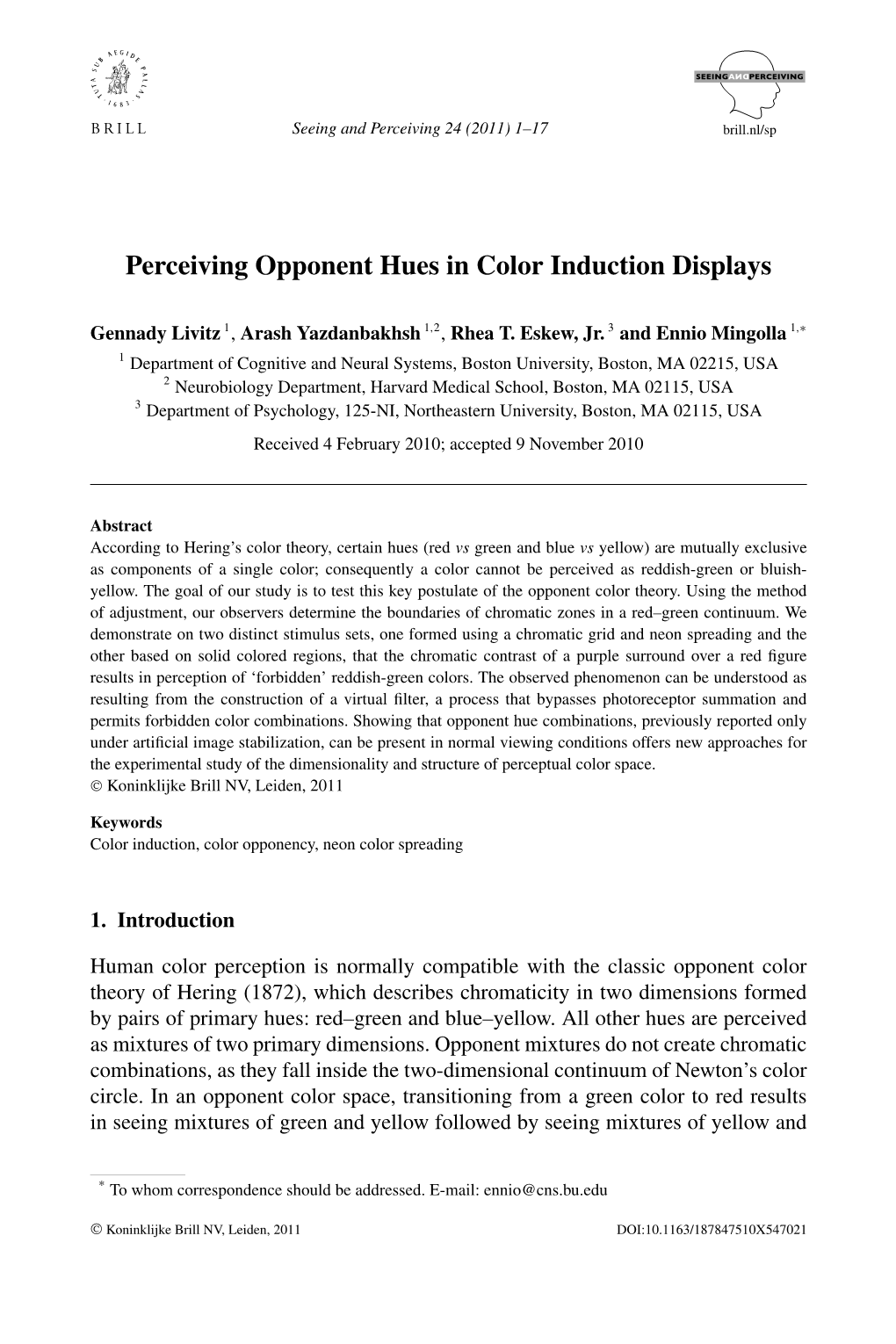
Load more
Recommended publications
-
On the Nature of Unique Hues
Reprinted from Dickinson, C., Murray, 1. and Carden, D. (Eds) 'John Dalton's Colour Vision Legacy', 1997, Taylor & Francis, London On the Nature of Unique Hues J. D. Mollon and Gabriele Jordan 7.1 .l INTRODUCTION There exist four colours, the Urfarben of Hering, that appear phenomenologically un- mixed. The special status of these 'unique hues' remains one of the central mysteries of colour science. In Hering's Opponent Colour Theory, unique red and green are the colours seen when the yellow-blue process is in equilibrium and when the red-green process is polarised in one direction or the other. Similarly unique yellow and blue are seen when the red-green process is in equilibrium and when the yellow-blue process is polarised in one direction or the other. Most observers judge that other hues, such as orange or cyan, partake of the qualities of two of the Urfarben. Under normal viewing conditions, however, we never experience mixtures of the two components of an opponent pair, that is, we do not experience reddish greens or yellowish blues (Hering, 1878). These observations are paradigmatic examples of what Brindley (1960) called Class B observations: the subject is asked to describe the quality of his private sensations. They differ from Class A observations, in which the subject is required only to report the identity or non- identity of the sensations evoked by different stimuli. We may add that they also differ from the performance measures (latency; frequency of error; magnitude of error) that treat the subject as an information-processingsystem and have been increasingly used in visual science since 1960. -
Unique Hues & Principal Hues
Received: 19 June 2018 Revised and accepted: 6 July 2018 DOI: 10.1002/col.22261 RESEARCH ARTICLE Unique hues and principal hues Mark D. Fairchild Program of Color Science/Munsell Color Science Abstract Laboratory, Rochester Institute of Technology, Integrated Sciences Academy, Rochester, This note examines the different concepts of encoding hue perception based on New York four unique hues (like NCS) or five principal hues (like Munsell). Various sources Correspondence of psychophysical and neurophysiological information on hue perception are Mark Fairchild, Rochester Institute of Technology, Integrated Sciences Academy, Program of Color reviewed in this context and the essential conclusion that is reached suggests there Science/Munsell Color Science Laboratory, are two types of hue perceptions being quantified. Hue discrimination is best quan- Rochester, NY 14623, USA. tified on scales based on five, equally spaced, principal hues while hue appearance Email: [email protected] is best quantified using a system based on four unique hues as cardinal axes. Much more remains to be learned. KEYWORDS appearance, discrimination, hue, Munsell 1 | INTRODUCTION note that the NCS system was designed to specify colors and their relations according to the character of their perception For over a century, the Munsell Color System has been used (appearance) while Munsell was set up to create scales of to describe color appearance, specify color relations, teach equal color discrimination, or color difference (rather than color concepts, and inspire new uses of color. It is unique in perception). Perhaps that distinction can be used to explain the sense that its hue dimension is anchored by five principal the difference in number of principal/cardinal hues. -
COLOUR11. Phenomenology
In a center- surround cell of any kind, you have two receptive fields, one the small center region, the other the surround region. In a chromatic center-surround field, each in innervated by one class of receptor, and — their signals are antagonistic + _ — If you took the input — + from 2 different cones, each with the same receptive field, and connected them to single neuron, you would have a neuron that signaled wavelength contrast (but not across any space.) — + — + _ — With a blue light in the surround, the surround provides inhibition. 100% blue surround inhibition; no center excitation. Entire cell = No firing (-100%) — + _ — With a blue light in the center, there is no excitation. Entire cell = no change to base rate — + _ — With a sky blue light in the surround, the surround provides inhibition (55%). With the sky blue light in the center, the center provides 10 % excitation Entire cell = - 40% — + _ — If only the surround is illuminated, then the surround provides inhibition (30%) Entire Cell: -30%. — + _ — If only the center is illuminated, then the center is excited by 30%. Entire Cell: +30%. — + _ — 1:1 ratio of excitation The Null Point: if both the center and the surround were illuminated by light at this wavelength, there would be no change to the base firing rate of the cell. Entire cell = Base rate firing — + _ — With green light in the center; the center is excited (45%). There is no light on the surround; so the surround provides no inhibition. Entire cell = +45% — + _ — With green light in surround inhibits the surround 10% There is no light on the center; so the center provides no excitation. -
Color for Philosophers: Unweaving the Rainbow
c o N T E N T 5 Foreword by Arthur Danto ix xv Preface xix Introduction I Color Perception and Science The physical causes of color 1 The camera and the eye 7 Perceiving lightness and darkness 19 26 Chromatic vision Chromatic response 36 The structure of phenomenal hues 40 Object metamerism, adaptation, and contrast 45 Some mechanisms of chromatic perception 52 II The Ontology of Color Objectivism 59 Standard conditions 67 Normal observers 76 Constancy and crudity 82 Ch romatic democracy 91 Sense data as color bearers 96 Materialist reduction and the illusion of color 109 III Phenomenology and Physiology THE RELATlONS OF COLORS TO EACH OTHER 113 T he resemblances of colors 113 The incompatibilities of colors 121 Deeper problems 127 OTHER MINDS 134 Spectral inversions and asymmetries 134 vii CONTENTS I nternalism and externalism 142 Other colors, other minds 145 COLOR LANGUAGE 155 Foci 155 The evolution of color categories 165 Boundaries and indeterminacy 169 Establishing boundaries 182 Color Plates following page 88 Appendix: Land's Retinex Theory of Color Vision 187 Notes 195 Glossary of Technical Terms 209 Further Reading 216 Bibliography 217 Acknowledgments 234 Indexes 237 viii F o R E w o R D Very few today still believe that philosophy is a disease of language and that its deliverances, due to disturbances of the grammatical un conscious, are neither true nor false but nonsense. But the fact re mains that, very often, philosophical theory stands to positive knowledge roughly in the relationship in which hysteria is said to stand to anatomical truth. -
Color-Opponent Mechanisms for Local Hue Encoding in a Hierarchical Framework
Color-opponent mechanisms for local hue encoding in a hierarchical framework Paria Mehrani,∗ Andrei Mouraviev,∗ Oscar J. Avella Gonzalez, John K. Tsotsos [email protected], [email protected], [email protected] , [email protected] Abstract L cone A biologically plausible computational model for color rep- M cone resentation is introduced. We present a mechanistic hier- archical model of neurons that not only successfully en- codes local hue, but also explicitly reveals how the con- tributions of each visual cortical layer participating in the process can lead to a hue representation. Our proposed model benefits from studies on the visual cortex and builds a network of single-opponent and hue-selective neurons. Local hue encoding is achieved through gradually increas- ing nonlinearity in terms of cone inputs to single-opponent cells. We demonstrate that our model's single-opponent neurons have wide tuning curves, while the hue-selective neurons in our model V4 layer exhibit narrower tunings, Cone responses as a function of x -4 -2 0 2 4 resembling those in V4 of the primate visual system. Our x simulation experiments suggest that neurons in V4 or later layers have the capacity of encoding unique hues. More- Figure 1: Spatial profile of a single-opponent L+M- cell. over, with a few examples, we present the possibility of The receptive field of this cells receives positive contri- spanning the infinite space of physical hues by combining butions from L cones and negative ones from M cones. the hue-selective neurons in our model. The spatial extent of these cone cells are different and Keywords| Single-opponent, Hue, Hierarchy, Visual determines the mechanism for this cell. -
The Abney Effect: Chromattcity Coordinates of Unique and Other Constant Hues
ON?-698931 Sj 00 - 0.00 CopyrIght ( 14X1 Pergxnon Press Lrd THE ABNEY EFFECT: CHROMATTCITY COORDINATES OF UNIQUE AND OTHER CONSTANT HUES S. A. BURSS.* A. E. ELSXER.* 3. POI~ORSY and V. C. SMITH The Eye Research Laboratories. The University of Chicago. 939 E. 57th Street. Chicago. IL 50637. U.S.;\. (Rechwl 2 Sqvremher I98_.1. in relired,form 16 Septrnrhrr 1953) ;\bstract-We compared unique and other constant hue loci measured at a fixed retinal iliuminan~e for the same observers. When expressed in Judd chromaticity coordinates. unique hue and constant hue data agreed. Unique blue loci were curved. and unique red and green loci were noncollinear. These data imply that unique hues are not a linear transformation of color matching functions. Linear models are onI> an approximation. even at a single luminance level. Color Vision Abney effect Unique hues IZ;TRODUCTION points for a yellow/blue mechanism. Many in- Constant hues are colors equal in hue, but different vestigators find or predict balance points of the with respect to another attribute. such as saturation. red/green opponent mechanism consistent with a For instance, two lights may appear the same hue, linear combination of cone fundamentals (e.g. Sch- e.g. blue, when one is a spectral light and the other riidinger. 1925; Judd, 1949; Hurvich and Jameson, is a mixture of two or more lights. Since mixtures of 1955; Judd and Yonemura. 1970; Larimer PI al., a chromatic light and an achromatic light often differ 1974; Raaijamakers and de Weert, 1974: Ingling et in hue as well as saturation (Abney, 1910), constant al., 1978a; Romeskie, 1978). -
A Critical Theory of Colour Concerning the Legality and Implications of Colour in Public Space
CONSUMING COLOUR: A CRITICAL THEORY OF COLOUR CONCERNING THE LEGALITY AND IMPLICATIONS OF COLOUR IN PUBLIC SPACE BY MICHELLE CORVETTE DEPARTMENT OF ART GOLDSMITHS UNIVERSITY OF LONDON DECEMBER 2015 / MAY 2016 1 DECLARATION OF AUTHORSHIP I, Michelle Corvette, declare that this thesis has been generated by me as the result of my own original research. Where information has been derived from other sources, I confirm that this has been indicated in the thesis. © 2016 Michelle Corvette 2 DEDICATION To my angel 3 ACKNOWLEDGEMENTS “We are like islands in the sea, separate on the surface but connected in the deep.” ~ William James There are many wonderful individuals to whom I owe a solid recognition of gratitude for helping me complete this thesis and the accompanying degree. I sincerely thank each and every one of them from my heart and may the blessing of support be returned twice fold upon them. Specifically, I would like to express deep appreciation to Goldsmiths College, University of London’s Art Department and Richard Noble for continued support and the opportunity to be part of an exceptional art community. To my supervisors, Andrea Phillips and Stephen Johnstone, for their time, generosity, and knowledge. To my viva committee members, Roger Burrows and Sherman Sam, for their adroitness, support, and erudition. To my upgrade committee members, Jules Davidoff and Mark Harris, for their assistance, suggestions, and expertise. To John Chilver, Suhail Malik, Michael Newman, Catherine Grant and Ian Hunt for their guidance and scholarship. To my family: Bruce & Emma Anderson, Bryce Anderson, Frida Catlo Corvette, and to my friends: Christina Stanhope, Joy, Eddie, & Taylor Yeh, Tommy Taylor, Sugarbush Sayavong, Josh, Mary, & Hannah McMurry, Mimi & Joe Holt, Kyoung Kim, Megumi Tsuchiya, Emily Rosamond, Nuno Ramalho, Francisco Lobo, Manuel Angel, Carolina Rito, Linda Stupart, Krista Clark, Ed Peston, and others for their kindness, foundation, and humanity. -
Emery 2017 Variations in Normal Color Vision.Pdf
Vision Research xxx (2017) xxx–xxx Contents lists available at ScienceDirect Vision Research journal homepage: www.elsevier.com/locate/visres Variations in normal color vision. VI. Factors underlying individual differences in hue scaling and their implications for models of color appearance ⇑ Kara J. Emery a, Vicki J. Volbrecht b, David H. Peterzell c, Michael A. Webster a,d, a Graduate Program in Integrative Neuroscience, University of Nevada, Reno, Reno, NV 89557, United States b Department of Psychology, Colorado State University, Fort Collins, CO 80523, United States c College of Psychology, John F. Kennedy University, Pleasant Hill, CA 94624, United States d Department of Psychology, University of Nevada, Reno, Reno, NV 89557, United States article info abstract Article history: Observers with normal color vision vary widely in their judgments of color appearance, such as the speci- Received 1 August 2016 fic spectral stimuli they perceive as pure or unique hues. We examined the basis of these individual dif- Received in revised form 2 December 2016 ferences by using factor analysis to examine the variations in hue-scaling functions from both new and Accepted 6 December 2016 previously published data. Observers reported the perceived proportion of red, green, blue or yellow in Available online xxxx chromatic stimuli sampling angles at fixed intervals within the LM and S cone-opponent plane. These proportions were converted to hue angles in a perceptual-opponent space defined by red vs. green and Keywords: blue vs. yellow axes. Factors were then extracted from the correlation matrix using PCA and Varimax Color rotation. These analyses revealed that inter-observer differences depend on seven or more narrowly- Color appearance Individual differences tuned factors. -
1 Opponent Processes in Colour Vision: What Can Afterimages
Opponent Processes In Colour Vision: What Can Afterimages Teach Us? Ruth Pauli Supervisor: Mike Harris March 2010 1 ABSTRACT To investigate afterimage colours, participants (n=14) formed afterimages by fixating on coloured stimuli in bright light for approximately eight seconds. They then chose one colour from a selection that most closely matched the afterimage they were seeing. Afterimages were found to be complementary to the stimulus colour, rather than corresponding to the opponent pairs. Consequently, the hypothesised opponent mechanisms should be revised to reflect the complementary nature of afterimages. N.B. For the purpose of assessment at the University of Birmingham, this research project was originally submitted as two separate assignments: a literature review (submitted September 2009) and a practical report (submitted March 2010). Both sections have been included here in their original form. Consequently, some of the material covered in the literature review is repeated in the introduction to the practical report. KEY OPPONENCY AFTERIMAGES COMPLEMENTARY OPPONENT GOETHE WORDS: THEORY COLOURS COLOURS 2 Literature Review The study of colour vision is as old as science itself. Already in ancient Greece, prominent philosophers had begun to investigate the phenomenon of colour. Plato believed that vision occurs because the eye sends ‘visual rays’ out into the world (Plato, cited at www.colorsystem.com). He identified four basic colours: white, black, red and ‘radiant’. White light (considered synonymous with daylight) allows the eye to extend its visual rays, while black (darkness) has the opposite effect. The third colour, red, is the colour of fire. Fire makes the eye produce tears, which cause objects to acquire ‘radiance’ and glow with different colours. -
The Language of Colour Wheels
Journal of Perceptual Imaging R 2(1): 010401-1–010401-12, 2019. c Society for Imaging Science and Technology 2019 What is the ``Opposite'' of ``Blue''? The Language of Color Wheels Neil A. Dodgson School of Engineering and Computer Science, Victoria University of Wellington, Wellington, New Zealand E-mail: [email protected] Abstract. A color wheel is a tool for ordering and understanding hue. Different color wheels differ in the spacing of the colors around the wheel. The opponent-color theory, Munsell’s color system, the standard printer’s primaries, the artist’s primaries, and Newton’s rainbow all present different variations of the color wheel. I show that some of this variation is owing to imprecise use of language, based on Berlin and Kay’s theory of basic color names. I also show that the artist’s color wheel is an outlier that does not match well to the technical color wheels because its principal colors are so strongly connected to the basic color names. c 2019 Society for Imaging Science and Technology. [DOI: 10.2352/J.Percept.Imaging.2019.2.1.010401] 1. INTRODUCTION Color wheels provide a way to describe the ordering of hue and, in some cases, to aid in understanding color mixing. The artist's color wheel (Figures1 and2), epitomized by Itten [23], is used extremely widely in teaching. Its primary colors are red, yellow, and blue. This is the color wheel that students meet in primary school. In this wheel, the opposite of blue Figure 1. An Itten color wheel with twelve hues. -
Unique Hues Are Not Invariant with Brief Stimulus Durations’
Perpmon Press Ltd 1’419. Rtntcd an Great Brttrm UNIQUE HUES ARE NOT INVARIANT WITH BRIEF STIMULUS DURATIONS’ Arriz~ L. NAGY’ University of Michigan (Received I8 Seprrmber 197s) Abstract-Unique hue loci were obtained from three observers at several intensity levels with a I-set stimulus flash and a 17 msec stimulus flash. With the one-second stimulus the three spectral unique hues were approximately invariant but unique red was not. With the I7 msec stimulus none of the unique hues is strictly invariant. These results are discussed in terms of their implications for the nature of the cone signal inputs to the opponent color mechanisms. IL\iTRODUCTION recently been provided by Krantz (1975) and Larimer et al. (1974, 19X), whose work revolves around the The relationship between uniques hues and invariant question of whether unique hues are invariant hues hues has long been of interest in the study of color and whether additions of hues unique with respect to perception. Unique hues are the “psychologically” the same mechanism (e.g. unique yellow and unique primary or “pure” hues, which do not appear to be blue, which both are unique with respect to the red/ compounds of other hues: red, green, yellow. and green mechanism) result in another unique hue. These blue. The term invariant hues refers to those wave- two properties follow from the assumptions of fengths or spectral composites whose perceived hue modem opponent colors theory (Jameson and Hur- does not vary with changes in stimulus intensity. It is vich, 1955; Krantz, 1975), which include: clear that the hue of most stimuli does vary with stimulus intensity (Purdy, 1931). -
Experiencing Sensation and Perception Page 6.1 Chapter 6: Color Vision
Experiencing Sensation and Perception Page 6.1 Chapter 6: Color Vision Chapter 6 Color Vision Chapter Outline: I. Introduction a. What is Color b. Color, Color Mixing, and Newton i. Additive Color Mixing ii. Subtractive Color Mixing iii. Effect of the Illuminant c. Color Matching Experiments II. The Trichromatic Theory and Color Matching a. Color Matching in a Monochromat b. Color Matching in a Dichromat c. Trichromacy d. The CIE Diagram e. The Number of Cones We Have f. Cones in Animals III. The Color Opponent Theory and Color Naming a. Limits to the Trichromatic Theory b. Color Opponency c. Resolution IV. Color Deficiency and Color Blindness a. Anomalous Trichromats b. Dichromats c. Other Types of Color Deficiency d. Application Issues with Color Deficiencies e. Frequencies of Color Deficiencies and Some Possible Implications V. Effects of Color a. Chromatic Adaptation: McCollough Effect b. Behnam’s Top and Fechner Colors c. Color Assimilation d. Color Filling-in VI. Summary Experiencing Sensation and Perception Page 6.2 Chapter 6: Color Vision Introduction Color enriches all of our experiences. The beauty of the sunset is often given as an example how color enhances our experience. I recall one sunset in Cape May, New Jersey, one of the many lovely regions of the Garden State. It is at the very southern tip of the state, and actually looks west across the Delaware Bay. They have a rather cute tradition that you have to experience, but for our purposes it brought my family to the shore to watch the sunset. The day was beautiful with the orange-red sun falling into dusky blue of the bay, with a bright orange streak going from sun to me.