Magnesium Diboride Josephson Junctions with Mgo and Native Oxide Barriers
Total Page:16
File Type:pdf, Size:1020Kb
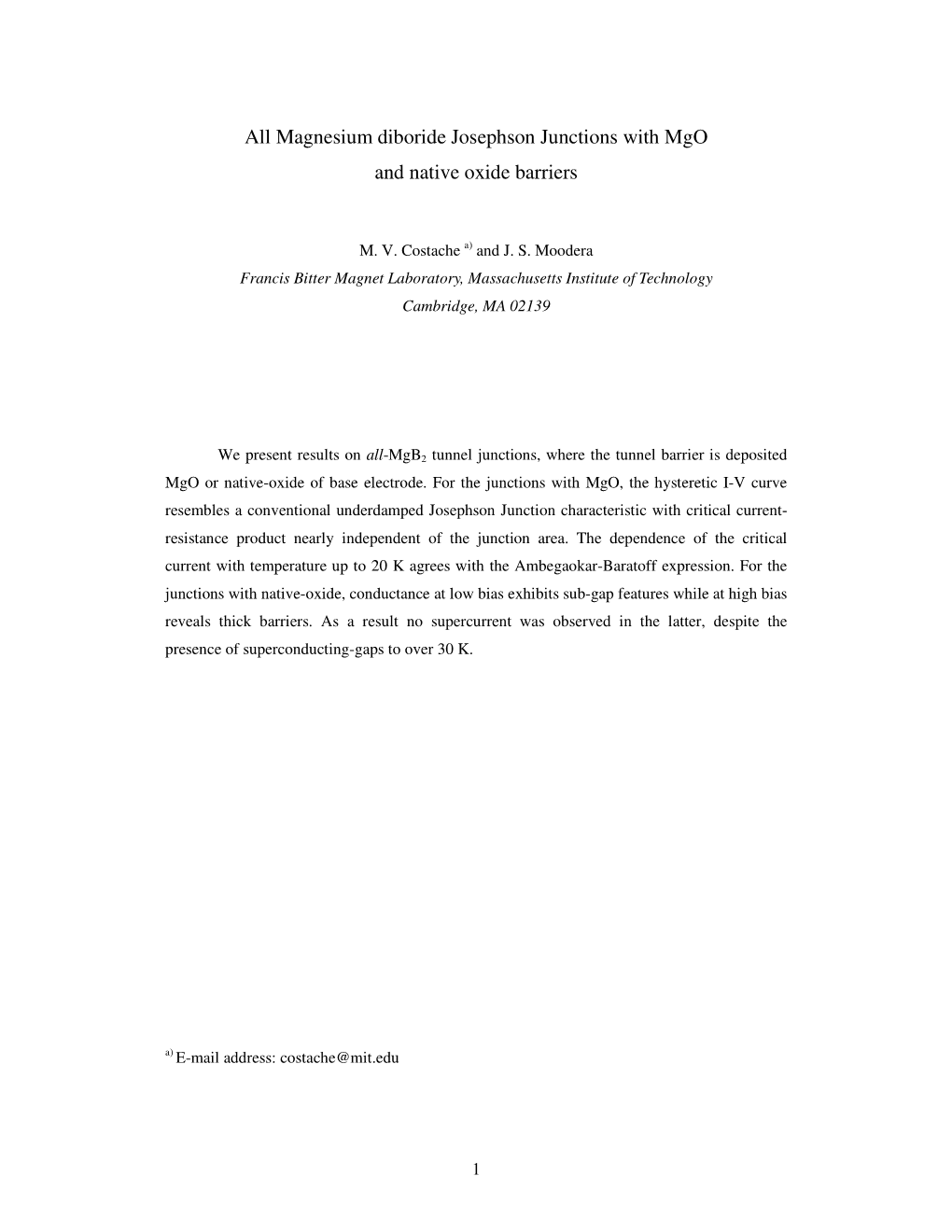
Load more
Recommended publications
-
Synthesis, Characterization and Energetic Performance
SYNTHESIS, CHARACTERIZATION AND ENERGETIC PERFORMANCE OF METAL BORIDE COMPOUNDS FOR INSENSITIVE ENERGETIC MATERIALS by Michael L. Whittaker A thesis submitted to the faculty of The University of Utah in partial fulfillment of the requirements for the degree of Master of Science Department of Materials Science and Engineering University of Utah May 2012 Copyright © Michael L. Whittaker 2012 All Rights Reserved The University of Utah Graduate School STATEMENT OF THESIS APPROVAL The thesis of Michael L. Whittaker has been approved by the following supervisory committee members: Raymond A. Cutler , Chair 03/09/2012 Date Approved Anil V. Virkar , Member 03/09/2012 Date Approved Gerald B. Stringfellow , Member 03/09/2012 Date Approved and by Feng Liu , Chair of the Department of Materials Science and Engineering and by Charles A. Wight, Dean of The Graduate School. ABSTRACT Six metal boride compounds (AlB2, MgB2, Al0.5Mg0.5B2, AlB12, AlMgB14 and SiB6) with particle sizes between 10-20 m were synthesized for insensitive energetic fuel additives from stoichiometric physical mixtures of elemental powders by high temperature solid state reaction. B4C was also investigated as a lower cost source of boron in AlB2 synthesis and showed promise as a boron substitute. Thermal analysis confirmed that the formation of boride compounds from physical mixtures decreased sensitivity to low temperature oxidation over the aluminum standard. Both Al+2B and AlB2 were much less sensitive to moisture degradation than aluminum in high humidity (10-100% relative humidity) and high temperature (20-80°C) environments. AlB2 was determined to be safe to store for extended periods of time in cool, dry environments. -
Comparing the Superconductivity of Mgb2 and Sr2ruo4
Comparing the superconductivity of MgB2 and Sr2RuO4 Etienne Palosa) (PHYS 232: Electronic Materials) The physical properties of two superconducting materials are compared in their normal and superconducting states. Their similarities and differences are reviewed in a systematic manner, beginning with a description of the unit cell. Structural, thermodynamic, electronic and magnetic properties are compared. In the super- conducting state, re compare the superconducting parameters of the materials and the effect on TC induced by physical or chemical changes to the systems. The sim- ilarities and differences between the conventional superconductor MgB2 and the unconventional superconductor Sr2RuO4 are discussed mainly within the context of experimental evidence reported in the literature. I. INTRODUCTION Some of the most important unresolved questions in condensed matter physics are re- lated to superconductivity. While the phenomena was observed over one-hundred years ago and an theory that explains conventional superconductivity has been established for the better half of a century, there is no unified theory of conventional and unconventional su- perconductivity. Or is there? However, through continuous theoretical, experimental and collaborative efforts, much has been learned about the physics of superconducting materi- als. The two main categories of superconductors are (i) conventional: those explained by the Bardeen{Cooper{Schrieffer (BCS) theory, and (ii) unconventional: materials whose su- perconducting mechanisms do not satisfy the conditions in BCS theory. In these materials, the superconducting mechanism is not phonon-mediated. In this work, a comparison of the physical properties of one conventional and one uncon- ventional superconductors is presented. On one hand, we have magnesium diboride, MgB2, a material whose superconductivity was discovered in 20011, decades after it's synthesis and crystal structure was first reported. -
Pressure-Induced Phase Transitions and Superconductivity In
www.nature.com/scientificreports OPEN Pressure-induced phase transitions and superconductivity in magnesium carbides Sooran Kim1,2, Kyoo Kim 1,3,7, Jahyun Koo4, Hoonkyung Lee 4, Byung Il Min1 & Duck Young Kim5,6* Crystal structure prediction and in silico physical property observations guide experimental synthesis in high-pressure research. Here, we used magnesium carbides as a representative example of computational high-pressure studies. We predicted various compositions of Mg–C compounds up to 150 GPa and successfully reproduced previous experimental results. Interestingly, our proposed MgC2 at high pressure >7 GPa consists of extended carbon bonds, one-dimensional graphene layers, and Mg atomic layers, which provides a good platform to study superconductivity of metal intercalated graphene nano-ribbons. We found that this new phase of MgC2 could be recovered to ambient pressure and exhibited a strong electron-phonon coupling (EPC) strength of 0.6 whose corresponding superconductivity transition temperature reached 15 K. The EPC originated from the cooperation of the out-of-plane and the in-plane phonon modes. The geometry confnement and the hybridization between the Mg s and C pz orbitals signifcantly afect the coupling of phonon modes and electrons. These results show the importance of the high-pressure route to the synthesis of novel functional materials, which can promote the search for new phases of carbon-based superconductors. Carbon has attracted much attention as a key element of contemporary science and technology. It is even con- sidered as the most promising platform for emergent energy materials, which might be able to replace the current main player, silicon, in the near future. -
Electronic Structure of Superconducting Graphite
Electronic structure of superconducting graphite intercalate compounds: The role of the interlayer state G´abor Cs´anyi, P. B. Littlewood, Andriy H. Nevidomskyy, C. J. Pickard and B. D. Simons Cavendish Laboratory, Madingley Road, Cambridge CB3 OHE, UK (Dated: October 29, 2018) arXiv:cond-mat/0503569v2 [cond-mat.supr-con] 12 Sep 2005 1 Although not an intrinsic superconductor, it has been long–known that, when intercalated with certain dopants, graphite is capable of exhibiting supercon- ductivity [1]. Of the family of graphite–based materials which are known to superconduct, perhaps the most well–studied are the alkali metal–graphite in- tercalation compounds (GIC) [2] and, of these, the most easily fabricated is the C8K system [3] which exhibits a transition temperature Tc ≃ 0.14 K [2]. By increasing the alkali metal concentration (through high pressure fabrication techniques), the transition temperature has been shown to increase to as much as 5K in C2Na [4]. Lately, in an important recent development, Weller et al. have shown that, at ambient conditions, the intercalated compounds C6Yb and C6Ca exhibit superconductivity with transition temperatures Tc ≃ 6.5 K and 11.5 K respectively [5], in excess of that presently reported for other graphite– based compounds. We explore the architecture of the states near the Fermi level and identify characteristics of the electronic band structure generic to GICs. As expected, we find that charge transfer from the intercalant atoms to the graphene sheets results in the occupation of the π–bands. Yet, remarkably, in all those – and only those – compounds that superconduct, we find that an interlayer state, which is well separated from the carbon sheets, also becomes occupied. -
Superconducting Properties of Graphene Doped Magnesium Diboride
10 Superconducting Properties of Graphene Doped Magnesium Diboride Xun Xu, Wenxian Li, Xiaolin Wang and Shi-Xue Dou University of Wollongong/ Institute for Superconducting and Electronic Materials Australia 1. Introduction Graphene, carbon in the form of monolayer sheets, has revealed astonishing and unique chemical and physical properties, which have made it an extremely active research topic in both materials science and physics (Novoselov, K. S. et al., 2004). Through chemical and materials integration, graphene is being actively exploited in a range of technological applications (Stankovich, S. et al., 2006). Superconductors can carry electrical current without any energy dissipation. The combination of both graphene and a superconductor into a composite has great potential for electrical devices and large scale applications. MgB2, a superconductor with a simple composition and two-gap feature has great potential for large current carrying applications, as demonstrated through a series of chemical dopings (Dou, S. X. et al. 2007). In the case of graphene’s, the strict two-dimensionality and its high electrical and thermal conductivities, make it an ideal candidate for integrating/doping into MgB2 in order to improve the superconducting properties. Substitutional chemistry can modify, in a controlled way, the electronic structures of superconductors and their superconducting properties, such as the transition temperature (Tc), critical current density (Jc), upper critical field (Hc2), and irreversibility field (Hirr). In particular, carbon containing dopants, including nano-meter sized carbon (nano-C), silicon carbide (SiC), carbon nanotubes (CNTs) and hydrocarbons/carbohydrates are effective means to enhance the Jc- field dependence and Hc2 (Ma, Y. et al., 2006, Senlowocz, B. -
Pressured-Induced Structural Phase Transition and Superconductivity in Nasn5
Pressured-induced structural phase transition and superconductivity in NaSn5 Chun-Mei Hao1, Yunguo Li1,2, Hong-Mei Huang1*, Yinwei Li1 and Yan-Ling Li1* 1. School of Physics and Electronic Engineering, Jiangsu Normal University, Xuzhou 221116, China 2. Department of Earth Sciences, UCL, Gower Street, London, WC1E 6BT, UK ABSTRACT: The structural and electronic properties of tin-rich compound NaSn5 were investigated under pressure up to 10 GPa based on the evolutionary algorithm (EA) technique coupled with first-principles total energy calculations. Upon compression, the known metallic tetragonal P-421m phase transforms into a metallic hexagonal P6/mmm phase at 1.85 GPa accompanied by an unusual change of existing form of Sn atoms. The P6/mmm phase can be interpreted as a quasi-layered sandwich structure with two Sn layers and one sodium layer. The presence of softening phonon modes and the existence of Fermi pockets together with the obvious Fermi surface nesting indicate a strong electron-phonon coupling (EPC) and thus potential superconductivity in P6/mmm phase. The strong EPC in P6/mmm phase is mainly attributed to the phonons from Sn1 atoms together with electrons from the Sn1-py and Sn1-pz states. The calculated superconducting critical temperature Tc of the P6/mmm phase is 5.91 K at 1.85 GPa. This study provides a new clue for designing intercalated compounds with superconductivity. 1 1. INTRODUCTION Study on the Na-Sn system started a century ago1, but the more concentrated study began in the 21st century. The study of phase formation and potential curves during sodium insertion has attracted many experimental and theoretical studies,2-5 because Na compounds with group IVA elements are considered to be a power source better than the widely used lithium-ion batteries (LIBS). -
Potential Application of Magnesium Diboride for Accelerator Magnet Applications*
Proceedings of the 2001 Particle Accelerator Conference, Chicago POTENTIAL APPLICATION OF MAGNESIUM DIBORIDE FOR ACCELERATOR MAGNET APPLICATIONS* L. D. Cooley, C. B. Eom, E. E. Hellstrom, and D. C. Larbalestier Applied Superconductivity Center, University of Wisconsin, Madison, Wisconsin 53706 USA Abstract flux pinning is lost and the critical current density Jc falls This paper reviews the potential of the newly discov- to zero. The maximum operating points for magnets are ered and unexpected 39 K superconductor MgB2 as a ~0.8H* because of the need to have finite Jc in the material for accelerator magnet strands. Unlike the high windings. The irreversibility fields of Nb-Ti, Nb3Sn and temperature cuprate superconductors, intergranular cur- MgB2 as a function of temperature are shown in fig. 1. rent flow in MgB2 is not obstructed by weak links, Due to the finite thermal energy of the magnetic flux-line removing one serious obstacle to the fabrication of wires. lattice, all three materials have H* 10-15% below Hc2. 5 2 The critical current density Jc exceeds 10 A/cm in 1 T Nb47Ti magnets can thus reach ~9 T at 4.2 K while field at 4.2 K and in self-field at 20 K. However, the Nb3Sn magnets can reach 20 to 22 T at 4.2 K. In compound is anisotropic and the perpendicular upper principle, HTS and Chevrel-phase conductors can reach critical field Hc2 in present bulk samples is comparable to still higher fields; for example, Bi-2212 conductors were or less than that in Nb47wt.%Ti at 4.2 K. -
Magnesium Diboride: Better Late Than Never
Magnesium Diboride: Better Late than Never With a superconducting transition temperature of 40 K and simple compound of two abundant, in- two superconducting gaps, MgB is full of surprises for both expensive elements. The long sought- 2 after, high-temperature, intermetallic experimentalists and theorists. superconductor had finally made its appearance—better late than never. Although the initial interest in Paul C. Canfield and George W. Crabtree MgB2 arose solely from its high Tc, fur- ther work revealed that MgB2 breaks he heyday of research into the basic properties of in- new ground for superconductivity based on the well-known Ttermetallic superconductors took place between 1950 electron–phonon interaction. The material displays a and 1980. During those years, the number of known su- plethora of remarkable features. MgB2 not only has im- perconducting intermetallic compounds (consisting of sev- portant technological potential (figure 1 and the cover of eral metallic and metalloid elements) grew explosively, this issue show images of MgB2 wire), but also will have a lasting impact on how the research community looks at and superconducting transition temperatures Tc were and looks for superconductors. pushed to just over 23 K (Nb3Ge). (In comparison, the first superconductor, discovered by Heike Kamerlingh Onnes in The understanding of this material has grown at a whirlwind rate, driven by the immediacy of preprint post- 1911, was mercury, with Tc = 4.15 K). Research groups all ings on the Internet. The daily appearance of new research over the world searched for higher and higher Tc values. The researchers were motivated by a basic desire to find results brought theory and experiment into resonance and an intrinsic limiting temperature for this intriguing quan- dramatically accelerated progress in the international tum phase and by a very applied interest in making use- community. -
Synthesis of Magnesium Diboride Superconductor with Cu Doping
SYNTHESIS OF MAGNESIUM DIBORIDE SUPERCONDUCTOR WITH CU DOPING A Thesis Presented to the Faculty of the Graduate School of Cornell University in Partial Fulfillment of the Requirements for the Degree of Master of Science by Shihao Tian January 2015 ⃝c 2015 Shihao Tian ALL RIGHTS RESERVED ABSTRACT For this thesis, we are going to explore the method of fabricating Magnesium Diboride (MgB2) superconductor using Magnesium and Boron powder with Copper doping. The composition and structural analysis of our products are examined by X-ray diffrac- tion(XRD) and Scanning Electron Microscopy(SEM). Later, we also perform resistance vs. temperature measurements on our products to find out the critical temperature. Based on the results of XRD and SEM, we believe we have successfully fabricated MgB2 with Cu doping. The successful products are fabricated using LabTemp furnace heated at 800◦C and 900◦C for 12 hours under argon environment. Also, the superconduct- ing test suggests our products possess high quality, and the superconducting transition temperature is found at Tc=39K. In the end, we discuss briefly about the possibility of making superconducting cavity employing our method. i ACKNOWLEDGEMENTS This project is supervised by Prof.Pierre Gourdain and Prof.Georg Hoffsatetter. Sincere thanks to my supervisors for their guidance and encouragement. Also, this work made use of the Cornell Center for Materials Research Shared Facilities which are supported through the NSF MRSEC program (DMR-1120296). ii Contents Abstract i Acknowledgements ii List of Figuresv -
Experimental Investigations of Magnesium Diboride Josephson Junctions
Experimental Investigations of Magnesium Diboride Josephson Junctions A Thesis Submitted to the Faculty of Drexel University by Steven A. Carabello in partial fulfillment of the requirements for the degree of Doctor of Philosophy May 2016 c Copyright 2016 Steven A. Carabello. This work is licensed under the terms of the Creative Commons Attribution-NonCommercial- NoDerivatives 4.0 International license. The license is available at http://creativecommons.org/licenses/by-nc-nd/4.0/. ii Acknowledgments As with any project spanning multiple years, I received help, encouragement, and support from many sources. The most direct help came from those who joined me in the ultra-low temperature laboratory at Drexel. I am especially thankful to have worked alongside Joey Lambert. We were a very effective team, and I am very grateful for his efforts. I also thank Dr. Roberto Ramos, for providing advice and support, pushes in the right direction, and for opening the world of condensed matter physics to me. Other students in the group, including Zechariah Thrailkill, Mariyan Stoyanov, Jerome Mlack, and Pubudu Galwaduge, made contributions without which I could not have achieved most of the results presented in this thesis. I am also very grateful to many others at Drexel. I thank the members of my committee for their service and support, and for keeping me on track: Dr. Bose, Dr. Barsoum, Dr. Penn, and Dr. Harb. Dr. Vogeley helped this part-time commuter student in maneuvering through all aspects of the physics graduate program. Maryann Fitzpatrick and Wolfgang Nadler were always available, encouraging, and able to help with hardware or technology of any kind. -
Effect of Pressure on the Superconducting Transition
Effect of pressure on the superconducting transition temperature of doped and neutron-damaged MgB2 S. L. Bud’ko, R. H. T. Wilke, M. Angst, and P. C. Canfield Ames Laboratory US DOE and Department of Physics and Astronomy, Iowa State University, Ames, IA 50011 Abstract Measurements of the superconducting transition temperatures for Al-doped, C- doped and neutron-damaged-annealed MgB2 samples under pressure up to ∼ 8 kbar are presented. The dTc/dP values change systematically with the decrease of the ambient pressure Tc in a regular fashion. The evolution of the pressure derivatives can be understood assuming that the change in phonon spectrum is a dominant contribution to dTc/dP . Key words: superconductors, high pressure, MgB2, doping PACS: 74.62.Fj, 74.62.Dh, 74.70.Ad The recently discovered [1] superconductivity, with a superconducting transi- tion temperature, Tc ∼ 40 K, in the simple binary intermetallic compound, MgB2, attracted a lot of attention to this material. Over the past few years a great deal of knowledge (phonon-mediated mechanism of superconductivity, high, temperature-dependent anisotropy of the upper critical field, two-gap nature of the superconducting state, etc.) was obtained about the pure MgB2 samples (see e.g. [2,3,4,5] for reviews). One of the approaches frequently used in attempts to understand the mechanism of superconductivity in a partic- ular material and, when possible, to improve its superconducting properties, arXiv:cond-mat/0411149v1 [cond-mat.supr-con] 5 Nov 2004 is to study material’s response to a small perturbation, like chemical substi- tution and/or pressure/strain. -
Ab Initio Study of the Electronic, Vibrational, and Mechanical Properties of the Magnesium Diboride Monolayer
Article Ab Initio Study of the Electronic, Vibrational, and Mechanical Properties of the Magnesium Diboride Monolayer Jelena Peši´c 1,* , Igor Popov 1,2, Andrijana Šolaji´c 1, Vladimir Damljanovi´c 1 , Kurt Hingerl 3, Milivoj Beli´c 4 and Radoš Gaji´c 1 1 Laboratory for graphene, other 2D materials and ordered nanostructures, Center for Solid State Physics and New Materials, Institute of Physics Belgrade, University of Belgrade, 11080 Belgrade, Serbia; [email protected] (I.P.); [email protected] (A.Š.); [email protected] (V.D.); [email protected] (R.G.) 2 Institute for Multidisciplinary Research, University of Belgrade, Kneza Višeslava 1, 11030 Belgrade, Serbia 3 Center for Surface and Nanoanalytics, Johannes Kepler University, 4040 Linz, Austria; [email protected] 4 Science Program, Texas A&M University at Qatar, P.O. Box 23874, Doha, Qatar; [email protected] * Correspondence: [email protected] Received: 15 March 2019; Accepted: 1 April 2019; Published: 2 April 2019 Abstract: Magnesium diboride gained significant interest in the materials science community after the discovery of its superconductivity, with an unusually high critical temperature of 39 K. Many aspects of the electronic properties and superconductivity of bulk MgB2 and thin sheets of MgB2 have been determined; however, a single layer of MgB2 has not yet been fully theoretically investigated. Here, we present a detailed study of the structural, electronic, vibrational, and elastic properties of monolayer MgB2, based on ab initio methods. First-principles calculations reveal the importance of reduction of dimensionality on the properties of MgB2 and thoroughly describe the properties of this novel 2D material.