Joint Workshop on New Technologies for Lunar
Total Page:16
File Type:pdf, Size:1020Kb
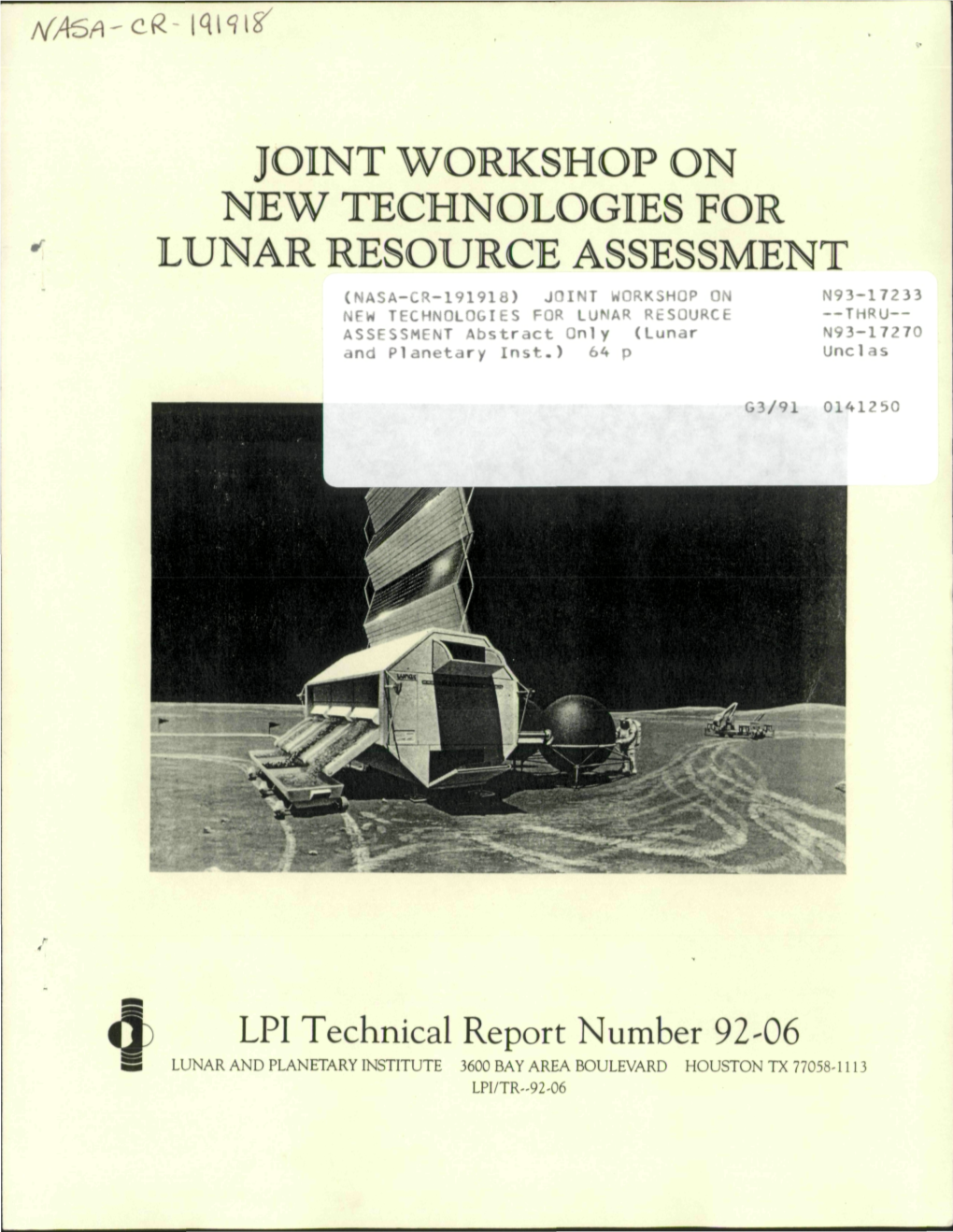
Load more
Recommended publications
-
Glossary Glossary
Glossary Glossary Albedo A measure of an object’s reflectivity. A pure white reflecting surface has an albedo of 1.0 (100%). A pitch-black, nonreflecting surface has an albedo of 0.0. The Moon is a fairly dark object with a combined albedo of 0.07 (reflecting 7% of the sunlight that falls upon it). The albedo range of the lunar maria is between 0.05 and 0.08. The brighter highlands have an albedo range from 0.09 to 0.15. Anorthosite Rocks rich in the mineral feldspar, making up much of the Moon’s bright highland regions. Aperture The diameter of a telescope’s objective lens or primary mirror. Apogee The point in the Moon’s orbit where it is furthest from the Earth. At apogee, the Moon can reach a maximum distance of 406,700 km from the Earth. Apollo The manned lunar program of the United States. Between July 1969 and December 1972, six Apollo missions landed on the Moon, allowing a total of 12 astronauts to explore its surface. Asteroid A minor planet. A large solid body of rock in orbit around the Sun. Banded crater A crater that displays dusky linear tracts on its inner walls and/or floor. 250 Basalt A dark, fine-grained volcanic rock, low in silicon, with a low viscosity. Basaltic material fills many of the Moon’s major basins, especially on the near side. Glossary Basin A very large circular impact structure (usually comprising multiple concentric rings) that usually displays some degree of flooding with lava. The largest and most conspicuous lava- flooded basins on the Moon are found on the near side, and most are filled to their outer edges with mare basalts. -
Chester County Marriages Bride Index 1885-1930
Chester County Marriages Bride Index 1885-1930 Bride's Last Name Bride's First Name Bride's Middle Bride's Date of Birth Bride's Age Groom's First Groom's Last Date of Application Date of Marriage Place of Marriage License # Dabney Ruth 47 Arthur Garner October 16, 1929 West Chester 29675 Dabundo Louise 18 Saverio DiMaio December 10, 1925 West Chester 26115.5 Dadley Fannie K 23 Albert Smith April 12, 1916 Toughkenamon 19118 Dagastina LorenzaFebruary 6, 1889 Michele Mastragiolo March 16, 1908 Norristown 13663 Dagne Eva EJuly 8, 1874 Jesse Downs December 27, 1899 West Chester 7490 Dagostina Philomena 18 Nicholas Tuscano August 2, 1925 Phoenixville 25847 D'Agostino Angelina 28 Gabriele Natale April 19, 1915 Norristown 18401 Dague Anna LSeptember 23, 1884 James Porter December 18, 1907 Parkesburg 13097 Dague CoraNovember 10, 1874 Vernon Powell February 10, 1904 Lionville 10244 Dague Lillie AApril 27, 1873 Frederick Gottier April 7, 1902 West Chester 9034 Dague M KatieJanuary 1, 1872 Charles Gantt April 17, 1900 Downington 7673 Dague Mary J 29 Ralph Young March 5, 1921 Coatesville 22856 Dague Sara Ellen 36 Rees Helms October 4, 1922 Honey Brook 23933 Dague Sarah Emma1858 James Eppihimer January 14, 1886 West Chester 104 Dahl Olga G 23 Claude Prettyman January 24, 1925 West Chester 25559 Dahms Elsie Annie 26 Chester Kirkhoff October 31, 1929 Pottstown 29710 Dailey Agnes1859 John McCarthy January 13, 1886 West Chester 084 Dailey Anna 19 Rhinehart Merkt August 14, 1913 Downingtown 17216 Dailey Anna RApril 29, 1877 18 Thomas Argne January 4, 1896 -
Martian Crater Morphology
ANALYSIS OF THE DEPTH-DIAMETER RELATIONSHIP OF MARTIAN CRATERS A Capstone Experience Thesis Presented by Jared Howenstine Completion Date: May 2006 Approved By: Professor M. Darby Dyar, Astronomy Professor Christopher Condit, Geology Professor Judith Young, Astronomy Abstract Title: Analysis of the Depth-Diameter Relationship of Martian Craters Author: Jared Howenstine, Astronomy Approved By: Judith Young, Astronomy Approved By: M. Darby Dyar, Astronomy Approved By: Christopher Condit, Geology CE Type: Departmental Honors Project Using a gridded version of maritan topography with the computer program Gridview, this project studied the depth-diameter relationship of martian impact craters. The work encompasses 361 profiles of impacts with diameters larger than 15 kilometers and is a continuation of work that was started at the Lunar and Planetary Institute in Houston, Texas under the guidance of Dr. Walter S. Keifer. Using the most ‘pristine,’ or deepest craters in the data a depth-diameter relationship was determined: d = 0.610D 0.327 , where d is the depth of the crater and D is the diameter of the crater, both in kilometers. This relationship can then be used to estimate the theoretical depth of any impact radius, and therefore can be used to estimate the pristine shape of the crater. With a depth-diameter ratio for a particular crater, the measured depth can then be compared to this theoretical value and an estimate of the amount of material within the crater, or fill, can then be calculated. The data includes 140 named impact craters, 3 basins, and 218 other impacts. The named data encompasses all named impact structures of greater than 100 kilometers in diameter. -
JPL to Map the Moon on India Mission
I n s i d e May 19, 2006 Volume 36 Number 10 News Briefs ............... 2 Griffin Visits Lab ............ 3 Special Events Calendar ...... 2 Passings, Letters ........... 4 Spitzer Sees Comet Breakup... 2 Retirees, Classifieds ......... 4 Jet Propulsion Laborator y A JPL state-of-the-art imaging spectrometer that will provide the first high-resolution spectral map of the JPL to entire lunar surface successfully completed its critical design review this week. The Moon Mineralogy Mapper, also known as “M3,” is one of two in- materials across the surface at high spatial resolution. This data map the struments that NASA is contributing to India’s first mission to the moon, will provide a much-needed long-term baseline for future exploration scheduled to launch in late 2007 or early 2008. By mapping the mineral activities. composition of the lunar surface, the mission will both provide clues to The mission’s observations will address several important scientific moon the early development of the solar system and guide future astronauts to issues, including early evolution of the solar system; fundamental stores of precious resources. processes acting on planets that shape their character; assessment of on India Chandrayaan-1 is India’s first deep-space mission as well as its first potential impact hazards to Earth; and assessment of space resources. lunar mission. “The entire M3 team feels honored to be able to partici- From its vantage point in orbit around the moon, the spacecraft will mission pate,” said Project Manager Tom Glavich of JPL. measure the sunlight reflected by all of the rocks and soil over which The instrument is well on its way to being delivered to the Chandray- it passes. -
Analysis of Polarimetric Mini-SAR and Mini-RF Datasets for Surface Characterization and Crater Delineation on Moon †
Proceeding Paper Analysis of Polarimetric Mini-SAR and Mini-RF Datasets for Surface Characterization and Crater Delineation on Moon † Himanshu Kumari and Ashutosh Bhardwaj * Photogrammetry and Remote Sensing Department, Indian Institute of Remote Sensing, Dehradun 248001, India; [email protected] * Correspondence: [email protected]; Tel.: +91-9410319433 † Presented at the 3rd International Electronic Conference on Atmospheric Sciences, 16–30 November 2020; Available online: https://ecas2020.sciforum.net/. Abstract: The hybrid polarimetric architecture of Mini-SAR and Mini-RF onboard Indian Chan- drayaan-1 and LRO missions were the first to acquire shadowed polar images of the Lunar surface. This study aimed to characterize the surface properties of Lunar polar and non-polar regions con- taining Haworth, Nobile, Gioja, an unnamed crater, Arago, and Moltke craters and delineate the crater boundaries using a newly emerged approach. The Terrain Mapping Camera (TMC) data of Chandrayaan-1 was found useful for the detection and extraction of precise boundaries of the cra- ters using the ArcGIS Crater tool. The Stokes child parameters estimated from radar backscatter like the degree of polarization (m), the relative phase (δ), Poincare ellipticity (χ) along with the Circular Polarization Ratio (CPR), and decomposition techniques, were used to study the surface attributes of craters. The Eigenvectors and Eigenvalues used to measure entropy and mean alpha showed distinct types of scattering, thus its comparison with m-δ, m-χ gave a profound conclusion to the lunar surface. The dominance of surface scattering confirmed the roughness of rugged material. The results showed the CPR associated with the presence of water ice as well as a dihedral reflection inside the polar craters. -
August 2017 Posidonius P & Luther
A PUBLICATION OF THE LUNAR SECTION OF THE A.L.P.O. EDITED BY: Wayne Bailey [email protected] 17 Autumn Lane, Sewell, NJ 08080 RECENT BACK ISSUES: http://moon.scopesandscapes.com/tlo_back.html FEATURE OF THE MONTH – AUGUST 2017 POSIDONIUS P & LUTHER Sketch and text by Robert H. Hays, Jr. - Worth, Illinois, USA March 5, 2017 01:28-01:48; UT, 15 cm refl, 170x, seeing 7-8/10. I drew these craters on the evening of March 4/5, 2017 while the moon was hiding some Hyades stars. This area is in northeast Mare Serenitatis west of Posidonius itself. Posidonius P is the largest crater on this sketch. The smaller crater south of P is Posidonius F and Posidonius G is the tiny pit to the north. There is a halo around Posidonius G, but this crater is noticeably north of the halo's center. A very low round swelling is northeast of Posidonius G. Luther is the crater well to the west of Posidonius P. All four of these craters are crisp, symmetric features, differing only in size. There are an assortment of elevations near Luther. The peak Luther alpha is well to the west of Luther, and showed dark shadowing at this time. All of the other features near Luther are more subtle than Luther alpha. One mound is between Luther and Luther alpha. Two more mounds are north of Luther, and a low ridge is just east of this crater. A pair of very low mounds are south of Luther. These are the vaguest features depicted here, and may be too conspicuous on the sketch. -
GRAIL Reveals Secrets of the Lunar Interior
GRAIL Reveals Secrets of the Lunar Interior — Dr. Patrick J. McGovern, Lunar and Planetary Institute A mini-flotilla of spacecraft sent to the Moon in the past few years by several nations has revealed much about the characteristics of the lunar surface via techniques such as imaging, spectroscopy, and laser ranging. While the achievements of these missions have been impressive, only GRAIL has seen deeply enough to reveal inner secrets that the Moon holds. LRecent Lunar Missions Country Name Launch Date Status ESA Small Missions for Advanced September 27, 2003 Ended with lunar surface impact on Research in Technology-1 (SMART-1) September 3, 2006 USA Acceleration, Reconnection, February 27, 2007 Extension of the THEMIS mission; ended Turbulence and Electrodynamics of in 2012 the Moon’s Interaction with the Sun (ARTEMIS) Japan SELENE (Kaguya) September 14, 2007 Ended with lunar surface impact on June 10, 2009 PChina Chang’e-1 October 24, 2007 Taken out of orbit on March 1, 2009 India Chandrayaan-1 October 22, 2008 Two-year mission; ended after 315 days due to malfunction and loss of contact USA Lunar Reconnaissance Orbiter (LRO) June 18, 2009 Completed one-year primary mission; now in five-year extended mission USA Lunar Crater Observation and June 18, 2009 Ended with lunar surface impact on Sensing Satellite (LCROSS) October 9, 2009 China Chang’e-2 October 1, 2010 Primary mission lasted for six months; extended mission completed flyby of asteroid 4179 Toutatis in December 2012 USA Gravity Recovery and Interior September 10, 2011 Ended with lunar surface impact on I Laboratory (GRAIL) December 17, 2012 To probe deeper, NASA launched the Gravity Recovery and Interior Laboratory (GRAIL) mission: twin spacecraft (named “Ebb” and “Flow” by elementary school students from Montana) flying in formation over the lunar surface, tracking each other to within a sensitivity of 50 nanometers per second, or one- twenty-thousandth of the velocity that a snail moves [1], according to GRAIL Principal Investigator Maria Zuber of the Massachusetts Institute of Technology. -
Asymmetric Terracing of Lunar Highland Craters: Influence of Pre-Impact Topography and Structure
Proc. L~lnorPli~nel. Sci. Corrf. /Of11 (1979), p. 2597-2607 Printed in the United States of America Asymmetric terracing of lunar highland craters: Influence of pre-impact topography and structure Ann W. Gifford and Ted A. Maxwell Center for Earth and Planetary Studies, National Air and Space Museum, Smithsonian Institition, Washington, DC 20560 Abstract-The effects of variable pre-impact topography and substrate on slumping and terrace for- mation have been studied on a group of 30 craters in the lunar highlands. These craters are charac- terized by a distinct upper slump block and are all situated on the rim of a larger, older crater or a degraded rim segment. Wide, isolated terraces occur where the rim of the younger crater coincides with a rim segment of the older crater. The craters are all located in Nectarianlpre-Nectarian highland units, and range in age from Imbrian to Copernican. A proposed model for formation of slump blocks in these craters includes the existence of layers with different competence in an overturned rim of the pre-existing crater. Such layering could have resulted from overturning of more coherent layers during formation of the Nectarian and pre-Nec- tarian craters. A combination of material and topographic effects is therefore responsible for terrace formation. Similar terrain effects may be present on other planets and should be considered when interpreting crater statistics in relation to morphology. INTRODUCTION Slumping, terracing or wall failure is an important process in formation and mod- ification of lunar craters. The process of slumping has been investigated by both geometrical (Cintala et a1 ., 1977) and theoretical models (Melosh, 1977; Melosh and McKinnon, 1979); however, these studies are dependent on morphologic constraints imposed by the geologic setting of the craters. -
Appendix I Lunar and Martian Nomenclature
APPENDIX I LUNAR AND MARTIAN NOMENCLATURE LUNAR AND MARTIAN NOMENCLATURE A large number of names of craters and other features on the Moon and Mars, were accepted by the IAU General Assemblies X (Moscow, 1958), XI (Berkeley, 1961), XII (Hamburg, 1964), XIV (Brighton, 1970), and XV (Sydney, 1973). The names were suggested by the appropriate IAU Commissions (16 and 17). In particular the Lunar names accepted at the XIVth and XVth General Assemblies were recommended by the 'Working Group on Lunar Nomenclature' under the Chairmanship of Dr D. H. Menzel. The Martian names were suggested by the 'Working Group on Martian Nomenclature' under the Chairmanship of Dr G. de Vaucouleurs. At the XVth General Assembly a new 'Working Group on Planetary System Nomenclature' was formed (Chairman: Dr P. M. Millman) comprising various Task Groups, one for each particular subject. For further references see: [AU Trans. X, 259-263, 1960; XIB, 236-238, 1962; Xlffi, 203-204, 1966; xnffi, 99-105, 1968; XIVB, 63, 129, 139, 1971; Space Sci. Rev. 12, 136-186, 1971. Because at the recent General Assemblies some small changes, or corrections, were made, the complete list of Lunar and Martian Topographic Features is published here. Table 1 Lunar Craters Abbe 58S,174E Balboa 19N,83W Abbot 6N,55E Baldet 54S, 151W Abel 34S,85E Balmer 20S,70E Abul Wafa 2N,ll7E Banachiewicz 5N,80E Adams 32S,69E Banting 26N,16E Aitken 17S,173E Barbier 248, 158E AI-Biruni 18N,93E Barnard 30S,86E Alden 24S, lllE Barringer 29S,151W Aldrin I.4N,22.1E Bartels 24N,90W Alekhin 68S,131W Becquerei -
Strategies for Detecting Biological Molecules on Titan
ASTROBIOLOGY Volume 18, Number 5, 2018 ª Mary Ann Liebert, Inc. DOI: 10.1089/ast.2017.1758 Strategies for Detecting Biological Molecules on Titan Catherine D. Neish,1 Ralph D. Lorenz,2 Elizabeth P. Turtle,2 Jason W. Barnes,3 Melissa G. Trainer,4 Bryan Stiles,5 Randolph Kirk,6 Charles A. Hibbitts,2 and Michael J. Malaska5 Abstract Saturn’s moon Titan has all the ingredients needed to produce ‘‘life as we know it.’’ When exposed to liquid water, organic molecules analogous to those found on Titan produce a range of biomolecules such as amino acids. Titan thus provides a natural laboratory for studying the products of prebiotic chemistry. In this work, we examine the ideal locales to search for evidence of, or progression toward, life on Titan. We determine that the best sites to identify biological molecules are deposits of impact melt on the floors of large, fresh impact craters, specifically Sinlap, Selk, and Menrva craters. We find that it is not possible to identify biomolecules on Titan through remote sensing, but rather through in situ measurements capable of identifying a wide range of biological molecules. Given the nonuniformity of impact melt exposures on the floor of a weathered impact crater, the ideal lander would be capable of precision targeting. This would allow it to identify the locations of fresh impact melt deposits, and/or sites where the melt deposits have been exposed through erosion or mass wasting. Determining the extent of prebiotic chemistry within these melt deposits would help us to understand how life could originate on a world very different from Earth. -
The Tsiolkovskiy Crater Landslide, the Moon: an LROC View
Icarus 337 (2020) 113464 Contents lists available at ScienceDirect Icarus journal homepage: www.elsevier.com/locate/icarus The Tsiolkovskiy crater landslide, the moon: An LROC view Joseph M. Boyce a,*, Peter Mouginis-Mark a, Mark Robinson b a Hawaii Institute for Geophysics and Planetology, University of Hawaii, Honolulu 96822, USA b School of Earth and Space Exploration, Arizona State University, Tempe, AZ 85281, USA ARTICLE INFO ABSTRACT Keywords: Evidence suggests that the lobate flow feature that extends ~72 km outward from the western rim of Tsiol Moon surface kovskiy crater is a long runout landslide. This landslide exhibits three (possibly four) morphologically different Landslides parts, likely caused by local conditions. All of these, plus the ejecta of Tsiolkovskiy crater, and its mare fill are Impact processes approximately of the same crater model age, i.e., ~3.55 � 0.1 Ga. The enormous size of this landslide is unique Geological processes on the Moon and is a result of a combination of several geometric factors (e.g., its location relative to Fermi Terrestrial planets crater), and that Tsiolkovskiy crater was an oblique impact that produced an ejecta forbidden zone on its western side (Schultz, 1976). The landslide formed in this ejecta free zone as the rim of Tsiolkovskiy collapsed and its debris flowedacross the relatively smooth, flatfloor of Fermi crater. In this location, it could be easily identified as a landslide and not ejecta. Its mobility and coefficientof friction are similar to landslides in Valles Marineris on Mars, but less than wet or even dry terrestrial natural flows.This suggests that the Mars landslides may have been emplaced dry. -
Summary of Sexual Abuse Claims in Chapter 11 Cases of Boy Scouts of America
Summary of Sexual Abuse Claims in Chapter 11 Cases of Boy Scouts of America There are approximately 101,135sexual abuse claims filed. Of those claims, the Tort Claimants’ Committee estimates that there are approximately 83,807 unique claims if the amended and superseded and multiple claims filed on account of the same survivor are removed. The summary of sexual abuse claims below uses the set of 83,807 of claim for purposes of claims summary below.1 The Tort Claimants’ Committee has broken down the sexual abuse claims in various categories for the purpose of disclosing where and when the sexual abuse claims arose and the identity of certain of the parties that are implicated in the alleged sexual abuse. Attached hereto as Exhibit 1 is a chart that shows the sexual abuse claims broken down by the year in which they first arose. Please note that there approximately 10,500 claims did not provide a date for when the sexual abuse occurred. As a result, those claims have not been assigned a year in which the abuse first arose. Attached hereto as Exhibit 2 is a chart that shows the claims broken down by the state or jurisdiction in which they arose. Please note there are approximately 7,186 claims that did not provide a location of abuse. Those claims are reflected by YY or ZZ in the codes used to identify the applicable state or jurisdiction. Those claims have not been assigned a state or other jurisdiction. Attached hereto as Exhibit 3 is a chart that shows the claims broken down by the Local Council implicated in the sexual abuse.