Mechanisms by Which Pentane and Hexane Adsorb on Silica Gel
Total Page:16
File Type:pdf, Size:1020Kb
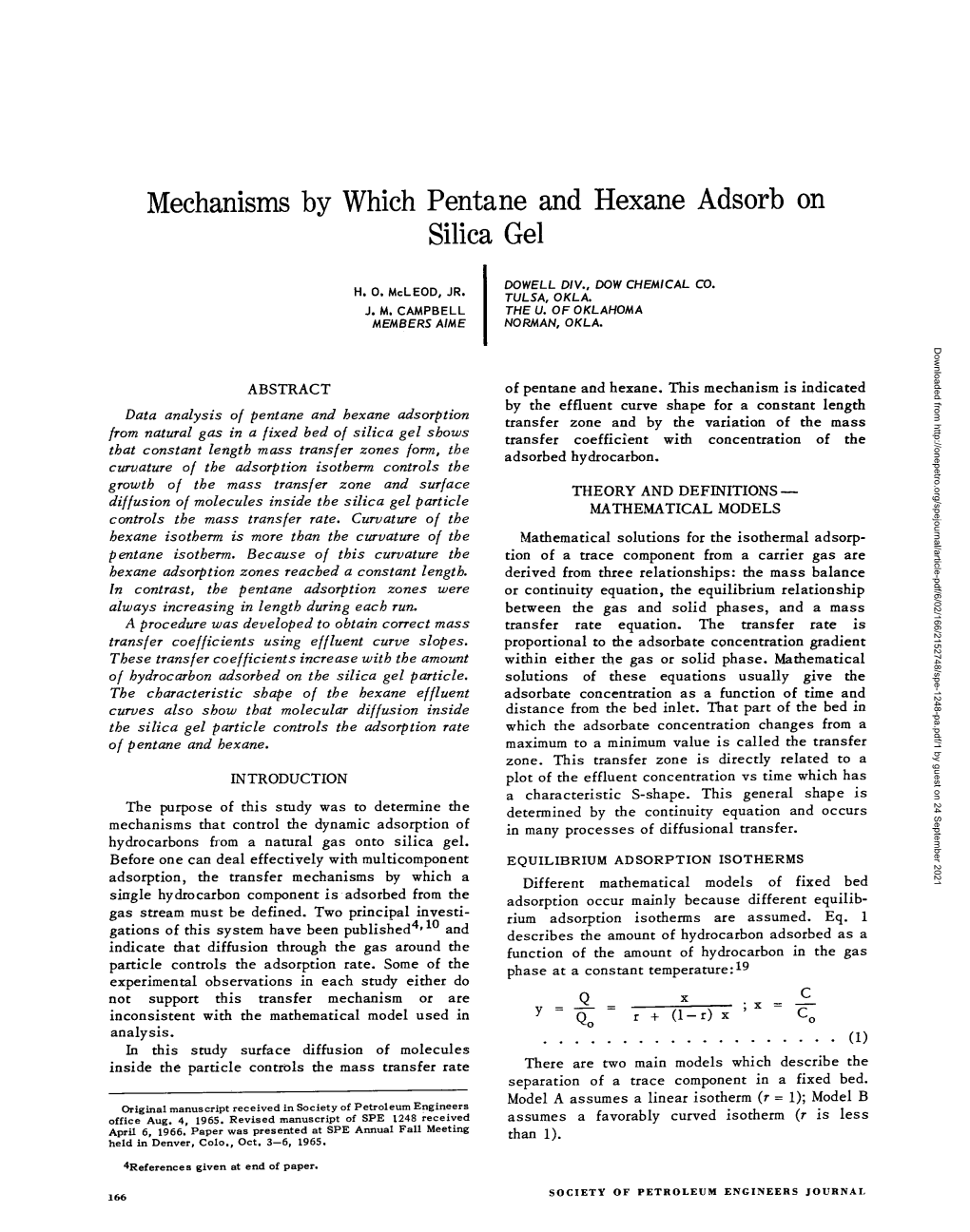
Load more
Recommended publications
-
Methane Hydrocarbon Compounds During Wintertime in Beijing
Atmos. Chem. Phys. Discuss., doi:10.5194/acp-2016-783, 2016 Manuscript under review for journal Atmos. Chem. Phys. Published: 16 December 2016 c Author(s) 2016. CC-BY 3.0 License. The levels, variation characteristics and sources of atmospheric non- methane hydrocarbon compounds during wintertime in Beijing, China Chengtang Liu1,3, Yujing Mu1,2 *, Junfeng Liu1,3, Chenglong Zhang1,3, Yuanyuan Zhang1,3, Pengfei 5 Liu1,3, Hongxing Zhang1,4 1Research Center for Eco-Environmental Sciences, Chinese Academy of Sciences, Beijing 100085, China 2Center for Excellence in Regional Atmospheric Environment, Institute of Urban Environment, Chinese Academy of Sciences, Xiamen 361021, China 3University of Chinese Academy of Sciences, Beijing 100085, China 10 4Beijing Urban Ecosystem Research Station, Beijing, 100085, China Correspondence to: Yujing Mu ([email protected]) Abstract. Atmospheric non-methane hydrocarbon compounds (NMHCs) were measured at a sampling site in Beijing city from 15 December 2015 to 14 January 2016 to recognize their pollution levels, variation characteristics and sources. Fifty- 15 three NMHCs were quantified and the proportions of alkanes, alkenes, acetylene and aromatics to the total NMHCs were 49.8% ~ 55.8%, 21.5% ~ 24.7%, 13.5% ~ 15.9% and 9.3% ~ 10.7%, respectively. The variation trends of the NMHCs concentrations were basically identical and exhibited remarkable fluctuation, which were mainly ascribed to the variation of meteorological conditions, especially wind speed. The diurnal variations of NMHCs in clear days exhibited two peaks during the morning and evening rush hours, whereas the rush hours’ peaks diminished or even disappeared in the haze days, 20 implying that the relative contribution of the vehicular emission to atmospheric NMHCs depended on the pollution status. -
Isopentane Ipt
ISOPENTANE IPT CAUTIONARY RESPONSE INFORMATION 4. FIRE HAZARDS 7. SHIPPING INFORMATION 4.1 Flash Point: -70°F C.C. 7.1 Grades of Purity: Research: 99.99%; pure: Common Synonyms Watery liquid Colorless Gasoline-like odor (approx.) 99.4%; technical: 97% 2-Methylbutane 4.2 Flammable Limits in Air: 1.4%-8.3% 7.2 Storage Temperature: Ambient Floats on water. Flammable, irritating vapor is produced. Boiling point 4.3 Fire Extinguishing Agents: Dry 7.3 Inert Atmosphere: No requirement chemical, foam, or carbon dioxide is 82°F. 7.4 Venting: Open (flame arrester) or pressure- 4.4 Fire Extinguishing Agents Not to Be vacuum Evacuate. Used: Water may be ineffective 7.5 IMO Pollution Category: C Keep people away. 4.5 Special Hazards of Combustion Wear goggles and self-contained breathing apparatus. Products: Not pertinent 7.6 Ship Type: 3 Shut off ignition sources and call fire department. 4.6 Behavior in Fire: Highly volatile liquid. 7.7 Barge Hull Type: Currently not available Avoid contact with liquid and vapor. Vapors may explode when mixed with air. Stay upwind and use water spray to ``knock down'' vapor. Notify local health and pollution control agencies. 4.7 Auto Ignition Temperature: 800°F 8. HAZARD CLASSIFICATIONS 4.8 Electrical Hazards: Not pertinent 8.1 49 CFR Category: Flammable liquid FLAMMABLE. Fire 4.9 Burning Rate: 7.4 mm/min. 8.2 49 CFR Class: 3 Flashback along vapor trail may occur. 4.10 Adiabatic Flame Temperature: Currently 8.3 49 CFR Package Group: I Vapor may explode if ignited in an enclosed area. -
Molecular Dynamics Simulation Studies of Physico of Liquid
MD Simulation of Liquid Pentane Isomers Bull. Korean Chem. Soc. 1999, Vol. 20, No. 8 897 Molecular Dynamics Simulation Studies of Physico Chemical Properties of Liquid Pentane Isomers Seng Kue Lee and Song Hi Lee* Department of Chemistry, Kyungsung University, Pusan 608-736, Korea Received January 15, 1999 We have presented the thermodynamic, structural and dynamic properties of liquid pentane isomers - normal pentane, isopentane, and neopentane - using an expanded collapsed atomic model. The thermodynamic prop erties show that the intermolecular interactions become weaker as the molecular shape becomes more nearly spherical and the surface area decreases with branching. The structural properties are well predicted from the site-site radial, the average end-to-end distance, and the root-mean-squared radius of gyration distribution func tions. The dynamic properties are obtained from the time correlation functions - the mean square displacement (MSD), the velocity auto-correlation (VAC), the cosine (CAC), the stress (SAC), the pressure (PAC), and the heat flux auto-correlation (HFAC) functions - of liquid pentane isomers. Two self-diffusion coefficients of liq uid pentane isomers calculated from the MSD's via the Einstein equation and the VAC's via the Green-Kubo relation show the same trend but do not coincide with the branching effect on self-diffusion. The rotational re laxation time of liquid pentane isomers obtained from the CAC's decreases monotonously as branching increas es. Two kinds of viscosities of liquid pentane isomers calculated from the SAC and PAC functions via the Green-Kubo relation have the same trend compared with the experimental results. The thermal conductivity calculated from the HFAC increases as branching increases. -
TCEQ Interoffice Memorandum
TCEQ Interoffice Memorandum To: Tony Walker Director, TCEQ Region 4, Dallas/Fort Worth Alyssa Taylor Special Assistant to the Regional Director, TCEQ Region 4, Dallas/Fort Worth From: Shannon Ethridge, M.S., D.A.B.T. Toxicology Division, Office of the Executive Director Date: Draft, 2014 Subject: Toxicological Evaluation of Results from an Ambient Air Sample for Volatile Organic Compounds Collected Downwind of the EagleRidge Energy, LLC - Woodland Estates West Unit (Latitude 32.595248, Longitude -97.160361) in Mansfield, Tarrant County, Texas Sample Collected on November 26, 2013, Request Number 1312003 (Lab Sample 1312003-001) Key Points • Reported concentrations of target volatile organic compounds (VOCs) were either not detected or were detected below levels of short-term health and/or welfare concern. Background On November 26, 2013, a Texas Commission on Environmental Quality (TCEQ) Region 4 air investigator collected a 30-minute canister sample (Lab Sample 1312003-001) downwind of the EagleRidge Energy, LLC - Woodland Estates West Unit (Latitude 32.595248, Longitude -97.160361) in Mansfield, Tarrant County, Texas. The sample was collected in response to a citizen complaint of a sore throat. The investigator did not experience an odor or health effects while sampling. Meteorological conditions measured at the site or nearest stationary ambient air monitoring site indicated that the ambient temperature was 44.3°F with a relative humidity of 63.6%, and winds were from the north (360°) at 7.5 to 9.5 miles per hour. The sampling site was less than 100 feet from the nearest possible emission source (tanks). The nearest location where the public could have access was approximately 301 to 500 feet from the possible emission source. -
Section 2. Hazards Identification OSHA/HCS Status : This Material Is Considered Hazardous by the OSHA Hazard Communication Standard (29 CFR 1910.1200)
SAFETY DATA SHEET Flammable Gas Mixture: Ethane / Isobutane / Isopentane / N-Butane / N-Pentane / Nitrogen / Propane Section 1. Identification GHS product identifier : Flammable Gas Mixture: Ethane / Isobutane / Isopentane / N-Butane / N-Pentane / Nitrogen / Propane Other means of : Not available. identification Product use : Synthetic/Analytical chemistry. SDS # : 008109 Supplier's details : Airgas USA, LLC and its affiliates 259 North Radnor-Chester Road Suite 100 Radnor, PA 19087-5283 1-610-687-5253 24-hour telephone : 1-866-734-3438 Section 2. Hazards identification OSHA/HCS status : This material is considered hazardous by the OSHA Hazard Communication Standard (29 CFR 1910.1200). Classification of the : FLAMMABLE GASES - Category 1 substance or mixture GASES UNDER PRESSURE - Compressed gas SPECIFIC TARGET ORGAN TOXICITY (SINGLE EXPOSURE) (Narcotic effects) - Category 3 AQUATIC HAZARD (LONG-TERM) - Category 3 GHS label elements Hazard pictograms : Signal word : Danger Hazard statements : Extremely flammable gas. Contains gas under pressure; may explode if heated. May form explosive mixtures in Air. May displace oxygen and cause rapid suffocation. May cause drowsiness and dizziness. Harmful to aquatic life with long lasting effects. Precautionary statements General : Read and follow all Safety Data Sheets (SDS’S) before use. Read label before use. Keep out of reach of children. If medical advice is needed, have product container or label at hand. Close valve after each use and when empty. Use equipment rated for cylinder pressure. Do not open valve until connected to equipment prepared for use. Use a back flow preventative device in the piping. Use only equipment of compatible materials of construction. Approach suspected leak area with caution. -
Analysis of Impurities in Ethylene by ASTM D6159-97
Petrochemical Applications Analysis of Impurities in Ethylene by ASTM D6159-97 Ethylene is one of the highest volume chemicals produced in the world, with global production exceed- ing 100 million metric tons annually. Ethylene is primarily used in the manufacture of polyethylene, ethylene oxide, and ethylene dichloride, as well as many other lower volume products. Most of these production processes use various catalysts to improve product quality and process yield. Impurities in ethylene can damage the catalysts, resulting in significant replacement costs, reduced product quality, process downtime, and decreased yield. Ethylene is typically manufactured through the use of steam cracking. In this process, gaseous or light liquid hydrocarbons are combined with steam and heated to 750–950°C in a pyrolysis furnace. Numerous free radical reactions are initiated and larger hydrocarbons are converted (cracked) into smaller hydro- carbons. The high temperatures used in steam cracking promote the formation of unsaturated or olefinic compounds like ethylene. Ethylene feedstocks must be tested to ensure that only high purity ethylene is delivered for subsequent chemical processing. Testing typically follows ASTM D6159-97, a GC/FID method which employs a two-column configura- tion consisting of an alumina PLOT column with KCl deactivation (50m x 0.53mm ID) coupled to a methyl silicone column (30m x 0.53mm ID x 5.0µm df). Figure 1 Methane and ethane are well resolved in high purity ethylene samples. Peak List 1. methane 2. ethane 3. ethylene GC_PC01109 Column: Rt®-Alumina BOND/KCl, 50m, 0.53mm ID, 10.0µm (cat.# 19760) in series with Rtx®-1, 30m, 0.53mm ID, 5.0µm (cat.# 10179), connected using a Universal Press-Tight® Connector (cat.# 20401) Sample: ethylene Inj.: 1µL split, 60mL/min. -
Rhea Van Gijzel
Eindhoven University of Technology MASTER Energy analysis and plant design for ethylene production from naphtha and natural gas van Gijzel, R.A. Award date: 2017 Link to publication Disclaimer This document contains a student thesis (bachelor's or master's), as authored by a student at Eindhoven University of Technology. Student theses are made available in the TU/e repository upon obtaining the required degree. The grade received is not published on the document as presented in the repository. The required complexity or quality of research of student theses may vary by program, and the required minimum study period may vary in duration. General rights Copyright and moral rights for the publications made accessible in the public portal are retained by the authors and/or other copyright owners and it is a condition of accessing publications that users recognise and abide by the legal requirements associated with these rights. • Users may download and print one copy of any publication from the public portal for the purpose of private study or research. • You may not further distribute the material or use it for any profit-making activity or commercial gain Process Engineering Multiphase Reactors group (SMR) Department of Chemical Engineering and Chemistry Den Dolech 2, 5612 AZ Eindhoven P.O. Box 513, 5600 MB Eindhoven The Netherlands www.tue.nl Graduation committee Prof. Dr. Ir. M. van Sint Annaland Dr. F. Gallucci Dr. V. Spallina (supervisor) Energy analysis and plant design for ethylene production Dr. T. Noël (external member) I. Campos Velarde from naphtha and natural gas Author R.A. van Gijzel MSc. -
HEAT of COMBUSTION of ISOBUTANE by Frederick D
U. S. DEPARTMENT OF COMMERCE NATIONAL BUREAU OF STANDARDS RESEARCH PAPER RP833 Part of Journal of Research of the National Bureau of Standards, Volume IS, October 1935 HEAT OF COMBUSTION OF ISOBUTANE By Frederick D. Rossini ABSTRACT The data of the present investigation yield for the heat evolved in the combus tion of gaseous isobutane in oxygen to form gaseous carbon dioxide and liquid water, at 25° C and a constant pressure of 1 atmosphere, the value 2871.06 ± 0. 53 international kiloj oules per mole. With the factor 1/4.1833, this value becomes 686,31 ± 0.13 kilocalories per mole. The isobutane used was estimated to be of such purity that its heat of combustion would differ from that of an a bsolutely pure sample by less than 0.01 percent. The only previous det ermination of the heat of combustion of isobutane was that made by Thomsen over 50 years ago on some impure material. From these new data values are computed for the heat of formation of isobut.ane. "Vhen combined with the previollsly reported datlt on the heat of combustion of normal butane, the present data yield for the reaction, n-C,H ID (gas) = iso C,HID (gn,s), at 25° C and 1 atmosphere, tJ.H= - 6. 82 ± 0.63 kilojoules, or - 1.63 ± 0.15 kilocalorics, pCI' mole. CONTENTS Page I. Introduction ____ __________ __ . ____ __ __ _____ _____ : __ .. _ _ _ __ _ _ _ _ 357 II. Units of energy, molecular weights, etc__ __________________ 3.58 III. Method, apparatus, and procedure ____ ___ ________________ . -
Selective Methylative Homologation: an Alternate Route to Alkane Upgrading John E
Published on Web 08/13/2008 Selective Methylative Homologation: An Alternate Route to Alkane Upgrading John E. Bercaw,‡ Nilay Hazari,‡ Jay A. Labinger,*,‡ Valerie J. Scott,‡ and Glenn J. Sunley*,# Arnold and Mabel Beckman Laboratories of Chemical Synthesis, California Institute of Technology, Pasadena, California 91125, and BP Chemicals Limited, Hull Research and Technology Centre, Kingston Upon Hull, North Humberside HU12 8DS, England Received April 24, 2008; E-mail: [email protected]; [email protected] Abstract: InI3 catalyzes the reaction of branched alkanes with methanol to produce heavier and more highly branched alkanes, which are more valuable fuels. The reaction of 2,3-dimethylbutane with methanol in the presence of InI3 at 180-200 °C affords the maximally branched C7 alkane, 2,2,3-trimethylbutane (triptane). With the addition of catalytic amounts of adamantane the selectivity of this transformation can be increased up to 60%. The lighter branched alkanes isobutane and isopentane also react with methanol to generate triptane, while 2-methylpentane is converted into 2,3-dimethylpentane and other more highly branched species. Observations implicate a chain mechanism in which InI3 activates branched alkanes to produce tertiary carbocations which are in equilibrium with olefins. The latter react with a methylating species generated from methanol and InI3 to give the next-higher carbocation, which accepts a hydride from the starting alkane to form the homologated alkane and regenerate the original carbocation. Adamantane functions as a hydride transfer agent and thus helps to minimize competing side reactions, such as isomerization and cracking, that are detrimental to selectivity. Introduction upgrading light hydrocarbons such as n-hexane to diesel- range linear alkanes.5,6 The catalytic conversion of abundant but relatively inert alkanes into higher value chemicals has been a longstanding The dehydrative condensation of methanol to hydrocarbons challenge for chemists and the petrochemical industry. -
Saturated Hydrocarbons
InterchApter F Saturated hydrocarbons Interference of light through a thin film of oil. Oil is composed of a complex mixture of hydrocarbons. The oil-derived fossil fuels propane, butane, and octane are examples of saturated hydrocarbons. University Science Books, ©2011. All rights reserved. www.uscibooks.com F. satUrAted hydrocArBonS F1 At one time chemists divided all compounds into two ganic compounds and their characteristic reactions. classes: inorganic and organic. Inorganic compounds We shall continue this introduction in the next two were classified as those that could be obtained from Interchapters, discussing unsaturated hydrocarbons minerals and other inanimate sources, whereas or- in Interchapter G and aromatic hydrocarbons, such ganic compounds were those obtained from living as benzene, in Interchapter H. We then look at the or once-living sources. For many years it was believed chemistry of some important classes of organic com- that organic compounds contained some sort of vital pounds in some of the later Interchapters; namely, force and that it was possible to synthesize organic alcohols, aldehydes, and ketones in Interchapter P, compounds only from other organic compounds, carboxylic acids in Interchapter R, and synthetic and which also contained this vital force. This belief, natural polymers in Interchapters S and T. called vitalism, was put to rest in 1829 when the German chemist Friedrich Wöhler synthesized the F-1. Alkanes Are hydrocarbons that contain organic compound urea, which occurs in urine, di- rectly from inorganic starting materials. Since then, only Single Bonds chemists have synthesized millions of organic com- Compounds that consist of only carbon and hydrogen pounds in the laboratory from simpler inorganic and atoms are called hydrocarbons. -
From Cracker to Key C5 Derivative Applications for Isoprene, DCPD and Piperylene Special Report Prospectus
IHS CHEMICAL C5 Value Chain Study: From Cracker to Key C5 Derivative Applications for Isoprene, DCPD and Piperylene Special Report Prospectus IHS Chemical Prospectus IHS CHEMICAL Contents Contents ..................................................................................................................................... 2 Introduction ................................................................................................................................. 3 What are C5 Petrochemicals? .............................................................................................. 3 What Industries do C5 Petrochemicals Supply? .................................................................. 4 Accessing C5 Petrochemicals .............................................................................................. 6 Issues Impacting the C5 Petrochemicals Industry ................................................................ 8 Study Objective .......................................................................................................................... 9 Study Scope ............................................................................................................................... 9 Market Dynamics for C5 Petrochemicals ............................................................................. 9 Pricing Dynamics for C5 Petrochemicals ............................................................................. 9 Downstream Industries Review ........................................................................................ -
Isopentane SAFETY DATA SHEET
SAFETY DATA SHEET Page: 1 of 6 Printed: 03/27/2020 Isopentane Revision: 03/27/2020 Supersedes Revision: 03/23/2015 1. PRODUCT AND COMPANY IDENTIFICATION Product Code: 00005 Product Name: Isopentane Company Name: Gas Innovations Phone Number: 18005 E. Hwy 225 +1 (281)471-2200 La Porte, TX 77571 Web site address: www.gasinnovations.com Emergency Contact: 3E (within United States) +1 (866)303-2640 Information: Infotrac (outside of United States) +1 (352)323-3500 2. HAZARDS IDENTIFICATION Specific Target Organ Toxicity (single exposure), Category 3 Aspiration Toxicity, Category 1 GHS Signal Word: Danger GHS Hazard Phrases: H304 - May be fatal if swallowed and enters airways. H336 - May cause drowsiness or dizziness. GHS Precautionary Phrases: P261 - Avoid breathing gas//vapors/spray. P271 - Use only outdoors or in a well-ventilated area. P273 - Avoid release to the environment. GHS Response Phrases: P301+310 - IF SWALLOWED: Immediately call a POISON CENTER or doctor/physician. P331 - Do NOT induce vomiting. P304+340 - IF INHALED: Remove victim to fresh air and keep at rest in a position comfortable for breathing. P312 - Call a POISON CENTER or doctor/physician if you feel unwell. P391 - Collect spillage. GHS Storage and Disposal P403+233 - Store container tightly closed in well-ventilated place. Phrases: P501 - Dispose of contents and containers in accordance with local, regional, national, and international regulations. Other Hazards: Toxic to aquatic life with long lasting effects. Potential Health Effects Isobutylene is a simple asphyxiant. Inhalation of high concentrations may cause rapid (Acute and Chronic): respiratory tract irritation, lightheadedness, central nervous system and depression in high concentrations.