Commissioning of Qualification of Structures, Systems and Components for Seismic and Environmental Loads of CIRENE Nuclear Power Plant
Total Page:16
File Type:pdf, Size:1020Kb
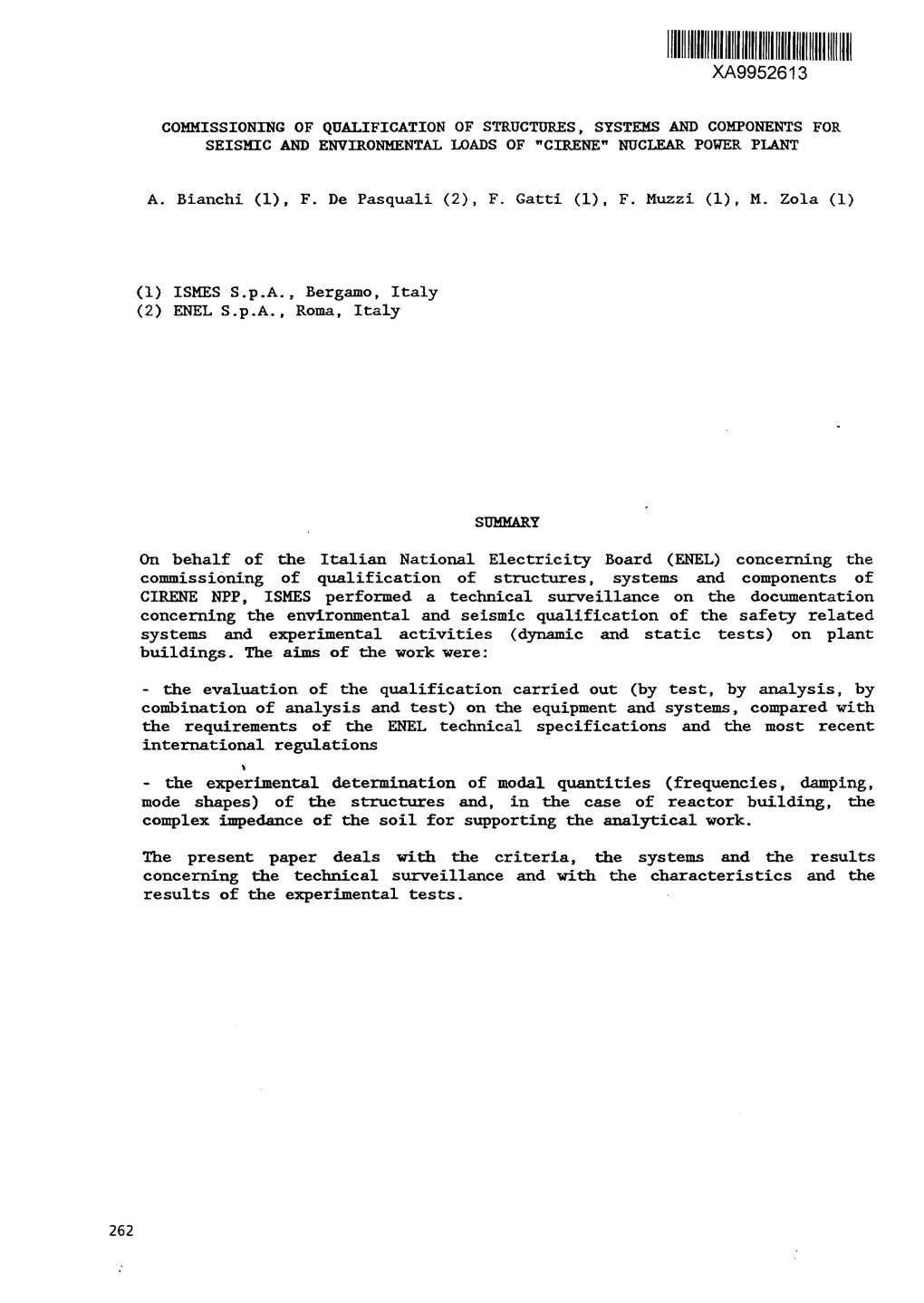
Load more
Recommended publications
-
Heavy Water Reactors: Status and Projected Development Designed in the Russian Federation
01-01915_TRS407.qxd 17.04.2002 14:04 Uhr Seite 1 Technical Reports Series No. This report commences with a review of the historical development of heavy water reactors (HWRs), detailing the various national efforts made in developing reactor concepts and taking them to the stage of prototype operation or commercial viability. Sections cover HWR economics, safety and fuel cycles. 4 0 7 Technical Reports Series No. 407 The future directions likely to be taken in the development of HWR technology are addressed through discussion of three national programmes: the Canadian CANDU design, the Advanced HWR currently under development in India, and an 'Ultimate Safe' reactor being Reactors: Status and Projected Development Heavy Water designed in the Russian Federation. Heavy Water Reactors: Status and Projected Development ISBN 92–0–111502–4 ISSN 0074–1914 €99.00 INTERNATIONAL ATOMIC ENERGY AGENCY, VIENNA, 2002 HEAVY WATER REACTORS: STATUS AND PROJECTED DEVELOPMENT The following States are Members of the International Atomic Energy Agency: AFGHANISTAN GREECE PARAGUAY ALBANIA GUATEMALA PERU ALGERIA HAITI PHILIPPINES ANGOLA HOLY SEE POLAND ARGENTINA HUNGARY PORTUGAL ARMENIA ICELAND QATAR AUSTRALIA INDIA REPUBLIC OF MOLDOVA AUSTRIA INDONESIA ROMANIA AZERBAIJAN IRAN, ISLAMIC REPUBLIC OF RUSSIAN FEDERATION BANGLADESH IRAQ SAUDI ARABIA BELARUS IRELAND SENEGAL BELGIUM ISRAEL SIERRA LEONE BENIN ITALY SINGAPORE BOLIVIA JAMAICA SLOVAKIA BOSNIA AND HERZEGOVINA JAPAN SLOVENIA BRAZIL JORDAN SOUTH AFRICA BULGARIA KAZAKHSTAN SPAIN BURKINA FASO KENYA SRI LANKA -
General Conference ENGLISH Original: ENGLISH, FRENCH
International Atomic Energy Agency GC(XI)/INF/97/Add.1 28 September 1967 GENERAL Distr. General Conference ENGLISH Original: ENGLISH, FRENCH ELEVENTH REGULAR SESSION PROGRESS IN PEACEFUL APPLICATIONS OF NUCLEAR ENERGY DURING THE YEAR 1966-67 Further Statements by Member States PROGRESS IN PEACEFUL APPLICATIONS OF JWUCLEAR ENERGY DURING THE YEAR 1966/67 1 CANADA The past year has been one of progress Another development in the past year was and expansion for the Canadian nuclear power the conclusion of an agreement between the programme. Probably the most significant develop Governments of India and Canada to build a ment was the decision by Canada's largest electric second 200-MW(e) CANDU unit at the Rajasthan utility company, the Hydro Electric Power Atomic Power Project in north-west India. The Commission of Ontario, to add two 540-megawatt first Rajasthan unit is under construction and is units to its Pickering nuclear power station, now expected to begin operation in 1970. In Pakistan being built near the City of Toronto. This will the Canadian General Electric Company Limited double the station's capacity to more than are building a 137-.\lW(e) heavy-water-moderated, 2000 MW(e) and make it the second largest natural-uranium-fuelled nuclear power plant. nuclear power plant under construction in North Completion is scheduled for 1970. America. Ontario Hydro's decision, involving an estimated outlay of $256 million, offers further confirmation of the confidence placed in the Thus there now are in operation or under nuclear power system developed by the Crown construction in Canada and abroad six nuclear company, Atomic Energy of Canada Limited. -
Atomic Energy of Canada Limited ADVANCED HWR POWER PLANTS
Atomic Energy of Canada Limited ADVANCED HWR POWER PLANTS DL-11O by W. BENNETT LEWIS Paper presented at the American Nuclear Society Power Division Conference Atlantic City, New Jersey 22-24 August, 1972 Chalk River Nuclear Laboratories Chalk River, Ontario November 1972 AECL-4304 PL-110 ATOMIC ENERGY OF CANADA LIMITED ADVANCED HWR POWER PLANTS by W. Bennett Lewis Paper presented at the American Nuclear Society Power Division Conference Atlantic City, New Jersey 22-24 August, 1972 Chalk River, Ontario November 1972 AECL-4304 DL-110 ADVANCED HWR POWER PLANTS1 by W. Bennett Lewis ABSTRACT Heavy-water reactors that are near-breeders can be alternatives for fast breeder reactors with the capability of meeting all the world's needs for power for many centuries. The Canada Deuterium Uranium (CANDU) and Steam-Generating Heavy-Water (SGHW) reactors are the only types of heavy-water reactor established for high-power generating stations. Those CANDU reactors now operating and under construction in large unit sizes are fuelled with natural uranium and cooled and moderated with D20, meeting the requirements of utilities desiring a minimum of enrichment together with an attractive capital cost. Possible modifications to design are the use of boiling light water as coolant, organic coolant, and thorium fuel cycles. Heavy-water reactors have a worldwide distribution. They can be classified as follows: 1) zero energy lattice and reactor physics research reactors; 2) high flux materials test, neutron beam and isotope production reactors; 3) engineering test reactors with high temperature loops; 4) demonstration power reactors; 5) prototype power reactors; and 6) commercial power reactors. -
Hybrid Systems, State of the Art and Prospects
— F. NER(; IA N(JC1.EARE HYBRID SYSTEMS STATE OF THE ART AND PROSPECTS Introd[~ction / The spallution process / Fissile material production concepts / Proposed transmutation concepts / Transm[{tation c(ipahility / Integral experiments / Safety analysis / Feasibility of Pu tr([r~,st?ll~t([tiot~ by hybrid systems / Conclusions .4 B(iCCAFURN1. P.A. LANDEYRO I INTRODUCTION the needs of experimental data to perform the projects in reasonably accurate conditions are discussed. The present work was started in the framework of the ENEA contribution to the Nuclear Energy Agency (NEA) Task Force on Physics Aspects of Trimsmuta- 2. THE SPALLATION PROCESS tion. Taking into account the present interest in the hy- Nuclear spa]lation occurs when a heavy target nu- brid systems technology. the paper was completed ad- cleus is hit by a medium energy particle such as a 1 ding the initial developments concerning the systems GeV proton (fig. I). Due to its high energy and cor- devoted to flssile material production, together with the respondingly small de Broglie wavelength, this proton more recent developments regarding systems dedicti- interacts with individual nuclei inside the nucleus, set- ted to electrical energy production. ting off a cascade of nucleon-nucleon collisions, i.e. In the last phase some parts of the original works the intranuclear cascade. In the course of this cascade, describing the systems were included in the text. some energetic nucleons may escape from the nucleus The hybrid (accelerator-subcritical reactor) systems and eventually hit another nucleus, thus giving rise to were thought to produce fissile material to avoid shor- an internuclear cascade. -
L%%LOSALAMOSSCIENTIFICLABORATORY Post Office Box 1663 Los Alamos
LA-7592-MS Informal Report ClC-l 4 REPORT COLLECTION REPRODUCTION COPY Production of Synthetic Gas from Nuclear Energy Sources .-Co C 5 0 Pre@red fcr the Texas Gas Transmission Corporation under > contract No. EW-78-Y-OM183 with the US Department of Energy. .- (43 5 .-> 5 L%%LOSALAMOSSCIENTIFICLABORATORY Post Office Box 1663 Los Alamos. New Mexico 87545 AXIAffrmstive Action/Equal Opwrtunity Employer TM. rcpucl was ptep.md .s an account of work sponsored by the Umted States Government. Nttthc. the Umtcd Slut- nor the Umled S1.1.s Dcpartmrnl of Energv. nor .W c.( Lhm CIWIIC.Y,,S. no, any of the,, cent,.clors. subcontr.cmrs. or lktlr ●mployees, m.kes any w.rr.n:y, txprest or wnpll.d. or .$sume$ .ny I.uI Ilabtl,ly m msponsibtltty for the acc. r.cy. completeness. y usefulness 0[ any information. atw. r.tus. product, or process disclosed, or represents th.t tu usewould “01 $nft,nne Iwivately owned mshls. UNITED STATES DEPARTMENT OF ENERGY CONTRACT W-740 B-ENG. 36 IA-7592-MS Informal Report Special Distribution Issued: April 1979 Production of Synthetic Gas from Nuclear Energy Sources C. A. Anderson J. C. Biery L. A. Booth L. M. Carruthers “K. E. COX F. T. Finch S. H. Nelson R. G. Palmer J. H. Pendergrass E. E. Stark J. K. Stutz Project Manager: E. E. Stark — -“F- ,. , ..-. CONTENTS 1. EXECUTIVE SUMMARY 1-1 (E. E. Stark) Introduction 1-1 Nuclear Fission 1-1 Introduction 1-1 Gas Core Reactor 1-2 High-Temperature Gas-Cooled Reactors (HTGRs) 1-3 Availability of Fuel for Fission Reactors 1-4 Fusion 1-5 Synthetic Gas Production Processes 1-6 Coal Gasification 1-6 Thermochemical Cycles 1-7 High-Temperature Electrolysis 1-8 Radiolysis 1-8 2. -
Comparative Prediction of Irradiation Test of Cnft and Cise Prototypes of Cirene Fuel Pins, a Prediction by Transuranus M1v1j12 Code
Comparative Prediction Of Irradiation Test Of Cnft And ISSN 0852-4777 Cise Prototypes Of Cirene Fuel Pins, A Prediction By Transuranus M1v1j12 Code (Suwardi) COMPARATIVE PREDICTION OF IRRADIATION TEST OF CNFT AND CISE PROTOTYPES OF CIRENE FUEL PINS, A PREDICTION BY TRANSURANUS M1V1J12 CODE Suwardi Center for Nuclear Fuel Technology - BATAN Tangerang Selatan -15310 Indonesia e-mail: [email protected] (Naskah diterima: 10-11-2013, disetujui: 29-01-2014) ABSTRACT COMPARATIVE PREDICTION OF IRRADIATION TEST OF CNFT AND CISE PROTOTYPES OF CIRENE FUEL PINS, A PREDICTION BY TRANSURANUS M1V1J12 CODE. A prototype of fuel pin design for HWR by CIRENE has been realized by Center for Nuclear Fuel Technology CNFT-BATAN. The prototype will be irradiated in PRTF Power Ramp Test (PRTF). The facility has been installed inside RSG-GA Siwabessy at Serpong. The present paper reports the preparation of experimentation and prediction of irradiation test. One previous PCI test report is found in, written by Lysell G and Valli G in 1973. The CNFT fuel irradiation test parameter is adapted to both PRTF and power loop design for RSG-GAS reactor in Serpong mainly the maxima of: rod length, neutrons flux, total power of rod, and power ramp rate. The CNFT CIRENE prototype design has been reported by Futichah et al 2007 and 2010. The AEC-India HWR fuel pin is of 19/22 fuel bundle design has also been evaluated as comparison. The first PCI test prediction has experiment comparison for Cise pin. The second prediction will be used for optimizing the design of ramp test for CNFT CIRENE fuel pin prototype. -
Euratom Bulletin of the European Atomic Energy Community : June 1965 Vol. IV No.2
uratom bulletin of the european atomic energy community june 1965 vol. IV no. 2 &m v£#V .'ft ;¥·.. -^ . *μ? ;% ^ Quarterly Information Bulletin of the Euro• pean Atomic Energy Community (Euratom) 1965-2 Contents: 34 Nuclear energy in Germany 44 Energy out of "pebbles" 49 What is ORGEL ?—A brief "recap" 50 How far have we got with the ORGEL project ? 54 CIRENE—A natural-uranium boiling- water reactor project 61 Euratom news: Nuclear energy from today until 2000—forecasts for the European Community; ORGEL—safety studies; A step towards nuclear superheat in water reactors; Production of uranium carbide single cristais at Ispra; Ways of obtaining higher burnups in graphite- gas reactors Published and edited by: Euratom, Dissemination of Information Directorate, 51-53 rue Be! I lard, Brussels 4. Telephone: 13 40 90 For subscription rates please see overleaf. Growth of o nuclear power plant—the Gund- remmingen "cactus" The Euratom Commission or any persons acting on its behalf disclaim all liability with respect to the completeness of the information contained in this periodical as well as to any damage which might result from the use of information disclosed or of equipment, methods or processes described therein. Any article published in this bulletin may be reproduced in whole or In part without restriction, provided that the source is mentioned. Picture credits: Page 2 of cover: AEG (Germany); page 35: Presse- u. Informa• tionsamt d. Bundesregierung, Bonn; Grodt- mann, Hamburg; page 39: KBB, Karlsruhe; page 40: Siemens; Linde; Erich Bauer, Karlsruhe; Interatom (Germany); page 41: AEG; page 43: Erich Bauer, Karlsruhe; page 48: Kernforschungsanlage Jülich; page 51 : Euratom JRC Ispra/Roberto Colom• bo; pages 52+53: Euratom JRC Ispra/ Ulrich Zimmermann; page 54: Roberto Zabban, Milan; page 60: Comm. -
Italian Committee for Nuclear Energy
NUCLEAR ENERGY IN ITALY By Carlo Salvetti * AbBtract 1969 was the final year of CNEN's - Italian Committee for Nuclear Energy - second Five-year Plan, in the course of which, the foundations were laid for the lines of nuclear development in the next decade, and even beyond it, CNEN'a principal programmes are the following! - CIIlLNE heavy water reactor programme within which a 35 MWe pressure tube heavy water moderated reactor prototype is under construction at Latina, The reactor will use natural or slightly enriched uranium and cooled by light water in a two-phase water/steam mixturej - the Fast Reactors Programme is finalized in the construction of PEC a fuel element testing reactor with a 116 MWt rated power and 140 MWt maximum power; - as the result of the collaboration between CHEN and the Italian Navy will be constructed the "Enrico Fermi" a logistic support ship of 18,000 t, powered by an 80 MWt PVffij - other CNEN reactor programme concerns ROVT organic moderated reactors for desalination with a capacity of fresh water per day from 50.000 to 200.000 ra.^s - as to fuel reprocessing activities two pilot plantB are in operation, namely fiUfiEX at Saluggia and ITREC at Trieaiaj - EUM.X 2 a full size industrial reprocessing plant, will be constructed in the near future - jointly by CNEN and Italian industry; - CNEN's activities refer also to research and development work for uranium enrichment plants, to fuel fabrication, to plutonium fuel development, to biology, to agriculture, to geomineralogy and high energy nuclear physics. ENEL - the Italian National Electricity Board - has recently awarded the contract for the fourth Italian Nuclear Plant, a 785 MWe BWR. -
The Optimal Placement of Shutoff Rods in CANDU Nuclear Reactors (Part A)
/ ~. ~., . THE OPTIMAL PLACEMENT OF SHUTOFF RODS IN CANDU NUCLEAR REACTORS by CHARLES W. GORDON, B.A.Sc. PART A: MCMASTER (OFF-CAMPUS) PROJECT* A Report Submitted to the School of Graduate Studies in Partial Fulfilment of the Requirements for the Degree Master of Engineering Department of Engineering Physics McMaster University Hamilton, Ontario, Canada November 1975 * One of two project reports: The other part is designated PART B: ON-CAMPUS PROJECT. MASTER OF ENGINEERING (1975) MCMASTER UNIVERSITY Department of Engineering Physics Hamilton, Ontario. TITLE: The Optimal Placement of Shutoff Rods in CANDU Nuclear Reactors AUTHOR: Charles W. Gordon, B.A.Sc. (Toronto) SUPERVISOR: Dr. M.H.M. Roshd NUMBER OF PAGES: viii, 68 i ABSTRACT The optimal placement of shutdown systems in power reactors is investigated, in particular, the placement of mechanical and liquid shut off rods. Two CANDU reactor cores were used as a basis for evaluation. The optimal shutdown system was defined here to be one which, with the least number of rods~ maximizes the reactivity depth of the system with the two most effective rods assumed to be absent. It was found that rows of rods placed parallel to the fuel channels were more effective and four of these rows were required in a simple core. For real cores where positions are limited six or seven rows ·were needed to obtain a large system worth. (Time analysis was not done to evaluate insertion rate and delay effects on the power transient in the case of an accident.) ii ACKNm'1LEDGEMENTS The author wishes to thank the staff of Atomic Energy of Canada Limited, Power Projects, for their assistance in the research for this project and, in particular, Dr. -
Small and Medium Power Reactors 1987
IAEA-TECDOC-445 SMAL MEDIUD LAN M POWER REACTORS 1987 A TECHNICAL DOCUMENT ISSUED BY THE INTERNATIONAL ATOMIC ENERGY AGENCY, VIENNA, 1987 SMAL MEDIUD LAN M POWER REACTORS 1987 IAEA, VIENNA, 1987 IAEA-TECDOC-445 Printed by the IAEA in Austria December 1987 PLEAS AWARE EB E THAT ALL OF THE MISSING PAGES IN THIS DOCUMENT WERE ORIGINALLY BLANK The IAEA does not normally maintain stocks of reports in this series. However, microfiche copie f thesso e reportobtainee b n sca d from IN IS Clearinghouse International Atomic Energy Agency Wagramerstrasse5 0 10 P.Ox Bo . A-1400 Vienna, Austria Orders should be accompanied by prepayment of Austrian Schillings 100, fore for e chequa th th f m IAEf m o n o n i i r eAo microfiche service coupons orderee whicb y hdma separately fro INIe mth S Clearinghouse. FOREWORD This TEC-DOC follows the publication of TEC DOC 347 Small and Medium Power Reactors Project Initiation Study - Phase I published in 1985 and TEC-DOC 376 Small and Medium Power Reactors 1985 published in 1986. It is mainly intended for decision makers in Developing Member States interested in embarking on a nuclear power programme. It consists of two parts : Part I; Guidelines for the introduction of Small and Medium Power Reactors in Developing Countries. These Guidelines were established during n Advisoro y Group Meeting hel n Vienni d5 May 1 ao t ,fro 1 19871 m . Their purpose is to review key aspects relating to the introduction f Smalo d Mediuan l m Power Reactor developinn i s g countries. -
Of Italian Civil Nuclear Programs, Beset by Lack of Strategic Planning, Exploitation for Personal Gain and Unscrupulous Political Conspiracies: 1946-1987
The “go-stop-go” of Italian civil nuclear programs, beset by lack of strategic planning, exploitation for personal gain and unscrupulous political conspiracies: 1946-1987 Angelo Baracca a, Giorgio Ferrari b and Roberto Renzetti c a Department of Physics, University of Florence; b Former ENEL specialist; c Physicist, www.fisicamente.net Angelo Baracca (corresponding author) Department of Physics and Astronomy, University of Florence via G. Sansone, 1 50019 Sesto Fiorentino (FI) Tel.: (39)3280196987 e-mail: [email protected] Giorgio Ferrari Former Enel nuclear expert Via A.Dulceri,171 00176 Roma Tel.: (39)064513698 e-mail: [email protected] Roberto Renzetti Physicist www.fisicamente.net Via Avegno 58 00165 Roma (RM) Tel (39)0666417178 e-mail: [email protected] Abstract The Italian civil nuclear projects had a very early origin, with the first ideas originating as far back as 1945. The construction of the first three plants dated back to the period 1956-1964, and at that time Italy ranked third in the world for installed power. However, the very ambitious projects were immediately after thwarted in the early 1960s by the “Ippolito trial”. Actually, a whole range of advanced programmes for the development of the country went to a stop, since they clashed with national and international major powers. Italy was relegated to a second rank power. The fourth nuclear plan was designed in 1970, and its commercial operation began in 1981. In the meantime, a strong anti-nuclear movement grew, and the position of the pro-nuclear Italian Communist Party began to change. After the Chernobyl accident, a national referendum was held, which in 1987 put an end to the Italian nuclear programmes. -
The Orgel Project 1959 - 1969
«»Mi ■ml 'f?npn mm wm- KN i ¡fe ¿••♦A Wà MfWWj * -liif \w. This document was prepared under the sponsorship of the Commission to«!*! of «he European Coronide, Pgjj^$|gH^B Neither the Commission of the European Communities, its contractors nor any person acting on their behalf : <■» make any warranty or representation, express or implied, with respect to the accuracy, completeness or usefulness of the information contained i Mí in this document, or that the use of any information, apparatus, method i y ri8hts!oror process disclosed in this document may not infringe privately owned m ggV «VttíÜ assume any liability with respect to the use of, or for damages resulting from the use of any information, apparatus, method or process disclose in this document. al m I*« Bö'1 i lb ι This report is on sale at the sses falli §& sted on cover page at the price of FF 16.60 FB 150. DM 11. Lit. 1.780 Fl. 11 When ordering, please quote the EUR number and the title which ivmñ indicated on the cover of each report. \m m w Printed by Van Muysewinkel, Brussels Luxembourg, September 1971 l*t»d ρΓ,ΐ' f' i ilijl This document was reproduced on the basis of them bes t ava 'li ÌH MJè& luì iWßh;« MJMÏ M "m ' l'I. Ufe •mmmWit ..••lii υ« «*Γ.Η· 1 m .-it •rt&UfflÆfel . ml.'MlilJhll l iivLÌil EUR 4505 f, e THE ORGEL PROJECT: 1959-1969 by J.C. LENY and S. ORLOWSKI Commission of the European Communities ORGEL Programme Joint Nuclear Research Centre - Ispra Establishment (Italy) Directorate of Heavy Water Reactors Luxembourg, September 1971 - 106 Pages - 14 Figures - B.Fr.