The Orchid Velamen
Total Page:16
File Type:pdf, Size:1020Kb
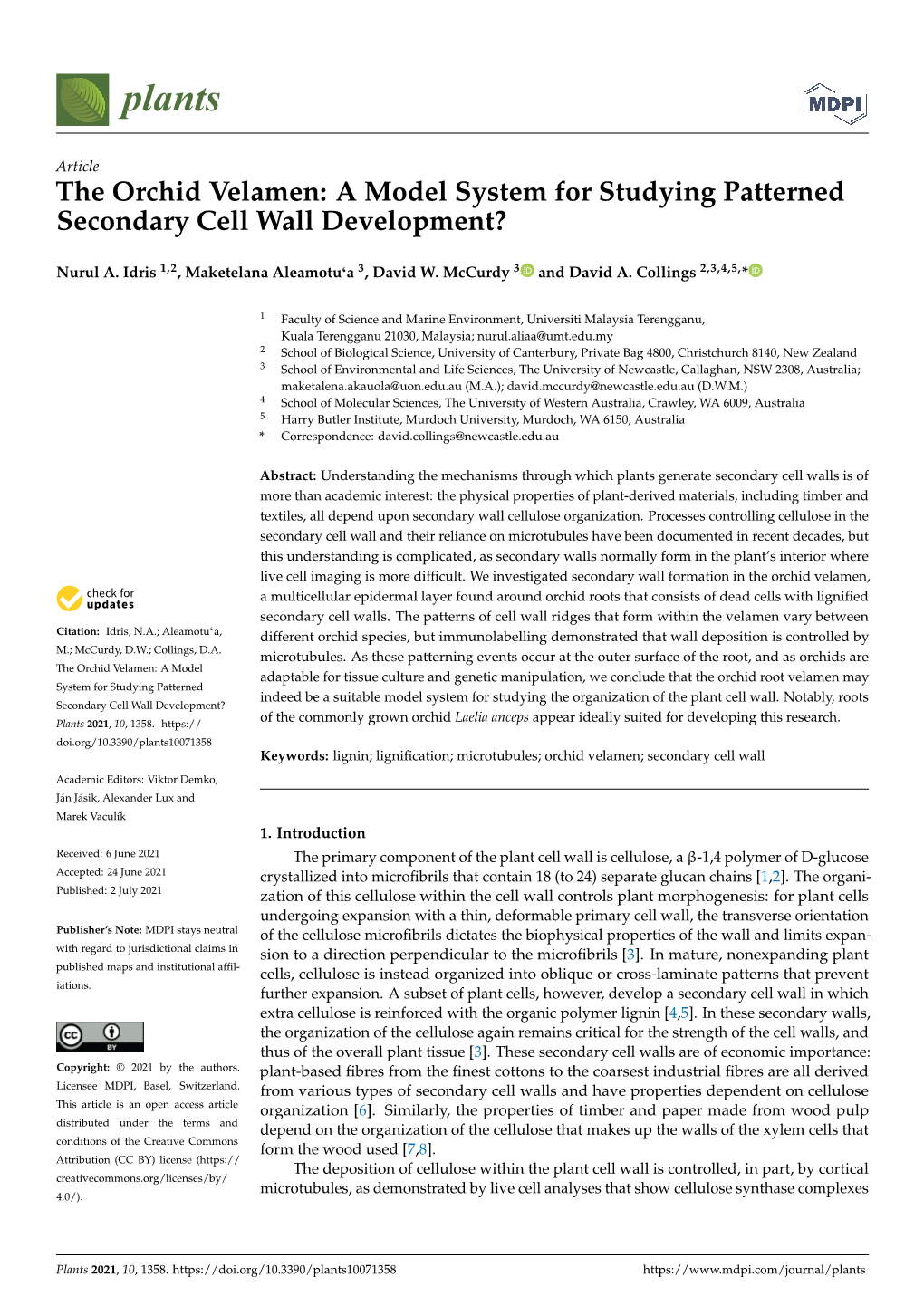
Load more
Recommended publications
-
February 1998 Newsletter
'i-.' ❖Odontoglossum Alliance^ Newsletter February 1998 Qdontoglossum Alliance Meeting The program for the Toronto meeting of the Southern Ontario Orchid i: Show has been mailed. If you did not receive on please contact: Peter Foot Box #241 Goodwood. Ontario LOG 1 AO 905-640-5643 905-640-0696 tFAXI The Odontoglossum Alliance annual meeting will be held Saturday, 9 May 1998 in Toronto, Canada. This will be held in conjunction with the Southern Ontario Orchid Show Orchid Show, 7-10 May 1998. This is the Mid-America Congress, Eastern Orchid Congress and the AOS Trustees meeting. The Odontoglossum Al liance program has been organized with the lectures beginning at 8:30 AM and continuing until noon. There are four lectures. Following the lectures will be a luncheon which will include a business meeting and an auc tion of fine and unusual Odontoglossum Alliance material. In addition we have arranged for an evening func tion at a Chinese restaurant in the same building as the lectures. The menu looks excellent. During the dinner we will also conduct an auction of fine Odontoglossum Alliance material. We will have divided the auction contributions between the lunch and dinner functions. The addition of a dinner will be a time to socialize with your Odontoglossum Alliance ffiends in a relaxed and enjoyable atmosphere. ■i. Both the lunch and dinner menus are printed at the end of this article. Also both the lunch and dinner are held in the same building as the lectures. Our thanks go to Marrio Ferrusi. who has made many of the arrangements. -
ORCHIDACEAE: ONCIDIINAE) and a SOLUTION to a TAXONOMIC CONUNDRUM Lankesteriana International Journal on Orchidology, Vol
Lankesteriana International Journal on Orchidology ISSN: 1409-3871 [email protected] Universidad de Costa Rica Costa Rica Dalström, Stig NEW COMBINATIONS IN ODONTOGLOSSUM (ORCHIDACEAE: ONCIDIINAE) AND A SOLUTION TO A TAXONOMIC CONUNDRUM Lankesteriana International Journal on Orchidology, vol. 12, núm. 1, abril, 2012, pp. 53-60 Universidad de Costa Rica Cartago, Costa Rica Available in: http://www.redalyc.org/articulo.oa?id=44339823005 How to cite Complete issue Scientific Information System More information about this article Network of Scientific Journals from Latin America, the Caribbean, Spain and Portugal Journal's homepage in redalyc.org Non-profit academic project, developed under the open access initiative LANKESTERIANA 12(1): 53—60. 2012. NEW COMBINATIONS IN ODONTOGLOSSUM (ORCHIDACEAE: ONCIDIINAE) AND A SOLUTION TO A TAXONOMIC CONUNDRUM STIG DALSTRÖM 2304 Ringling Boulevard, unit 119, Sarasota FL 34237, U.S.A. Research Associate: Lankester Botanical Garden, University of Costa Rica and Andean Orchids Research Center, University Alfredo Pérez Guerrero, Ecuador National Biodiversity Centre, Serbithang, Thimphu, Bhutan [email protected] ABSTRACT. The diminutively flowered Oncidium koechliniana demonstrates a unique combination of features that justifies a transfer of it and all here accepted species in closely related genera Cochlioda and Solenidiopsis to Odontoglossum, which is executed here. Distinguishing features to separate Odontoglossum from Oncidium are based on geographic distribution, and flower morphology, which is demonstrated with illustrations. RESUMEN. Oncidium koechliniana, de flores diminutas, presenta una combinacíon de características únicas que justifica su transferencia, así como de todas las especies aquí aceptadas de los génerosCochlioda y Solenidiopsis a Odontoglossum, transferencias que se hacen en este artículo. La características distintiva para separar Odontoglossum de Oncidium están basadas en distribución geográfica y morfología floral, que se muestran a través de ilustraciones. -
Redalyc.GENERIC RELATIONSHIPS of ZYGOPETALINAE (ORCHIDACEAE: CYMBIDIEAE): COMBINED MOLECULAR EVIDENCE
Lankesteriana International Journal on Orchidology ISSN: 1409-3871 [email protected] Universidad de Costa Rica Costa Rica WHITTEN, W. MARK; WILLIAMS, NORRIS H.; DRESSLER, ROBERT L.; GERLACH, GÜNTER; PUPULIN, FRANCO GENERIC RELATIONSHIPS OF ZYGOPETALINAE (ORCHIDACEAE: CYMBIDIEAE): COMBINED MOLECULAR EVIDENCE Lankesteriana International Journal on Orchidology, vol. 5, núm. 2, agosto, 2005, pp. 87- 107 Universidad de Costa Rica Cartago, Costa Rica Available in: http://www.redalyc.org/articulo.oa?id=44339808001 How to cite Complete issue Scientific Information System More information about this article Network of Scientific Journals from Latin America, the Caribbean, Spain and Portugal Journal's homepage in redalyc.org Non-profit academic project, developed under the open access initiative LANKESTERIANA 5(2):87-107. 2005. GENERIC RELATIONSHIPS OF ZYGOPETALINAE (ORCHIDACEAE: CYMBIDIEAE): COMBINED MOLECULAR EVIDENCE W. MARK WHITTEN Florida Museum of Natural History, University of Florida, Gainesville, FL 32611-7800, USA NORRIS H. WILLIAMS1 Florida Museum of Natural History, University of Florida, Gainesville, FL 32611-7800, USA ROBERT L. DRESSLER2 Florida Museum of Natural History, University of Florida, Gainesville, FL 32611-7800, USA GÜNTER GERLACH Botanischer Garten München Nymphenburg, Menzinger Str. 65. 80638 München, Germany FRANCO PUPULIN Jardín Botánico Lankester, Universidad de Costa Rica, P.O. Box 1031-7050 Cartago, Costa Rica 1Author for correspondence: orchid@flmnh.ufl.edu 2Missouri Botanical Garden, P.O. Box 299, St. Louis, Missouri 63166-0299, U.S.A. Mailing address: 21305 NW 86th Ave., Micanopy, Florida 32667. ABSTRACT. The phylogenetic relationships of the orchid subtribe Zygopetalinae were evaluated using parsimony analyses of combined DNA sequence data of nuclear ITS 1 and 2 (including the 5.8s region and portions of the flanking 18s and 26s regions) and of the plastid trnL intron plus the trnL-F intergenic spacer and the plastid matK. -
The Orchid Flora of the Colombian Department of Valle Del Cauca Revista Mexicana De Biodiversidad, Vol
Revista Mexicana de Biodiversidad ISSN: 1870-3453 [email protected] Universidad Nacional Autónoma de México México Kolanowska, Marta The orchid flora of the Colombian Department of Valle del Cauca Revista Mexicana de Biodiversidad, vol. 85, núm. 2, 2014, pp. 445-462 Universidad Nacional Autónoma de México Distrito Federal, México Available in: http://www.redalyc.org/articulo.oa?id=42531364003 How to cite Complete issue Scientific Information System More information about this article Network of Scientific Journals from Latin America, the Caribbean, Spain and Portugal Journal's homepage in redalyc.org Non-profit academic project, developed under the open access initiative Revista Mexicana de Biodiversidad 85: 445-462, 2014 Revista Mexicana de Biodiversidad 85: 445-462, 2014 DOI: 10.7550/rmb.32511 DOI: 10.7550/rmb.32511445 The orchid flora of the Colombian Department of Valle del Cauca La orquideoflora del departamento colombiano de Valle del Cauca Marta Kolanowska Department of Plant Taxonomy and Nature Conservation, University of Gdańsk. Wita Stwosza 59, 80-308 Gdańsk, Poland. [email protected] Abstract. The floristic, geographical and ecological analysis of the orchid flora of the department of Valle del Cauca are presented. The study area is located in the southwestern Colombia and it covers about 22 140 km2 of land across 4 physiographic units. All analysis are based on the fieldwork and on the revision of the herbarium material. A list of 572 orchid species occurring in the department of Valle del Cauca is presented. Two species, Arundina graminifolia and Vanilla planifolia, are non-native elements of the studied orchid flora. The greatest species diversity is observed in the montane regions of the study area, especially in wet montane forest. -
Oncidiinae: Orchidaceae) from PERU Lankesteriana International Journal on Orchidology, Vol
Lankesteriana International Journal on Orchidology ISSN: 1409-3871 [email protected] Universidad de Costa Rica Costa Rica Dalström, Stig; Deburghgraeve, Guido; Ruíz Perez, Saul THREE NEW SHOWY BUT ENDANGERED CYRTOCHILUM SPECIES (Oncidiinae: Orchidaceae) FROM PERU Lankesteriana International Journal on Orchidology, vol. 12, núm. 2, agosto, 2012, pp. 93- 99 Universidad de Costa Rica Cartago, Costa Rica Available in: http://www.redalyc.org/articulo.oa?id=44339824001 How to cite Complete issue Scientific Information System More information about this article Network of Scientific Journals from Latin America, the Caribbean, Spain and Portugal Journal's homepage in redalyc.org Non-profit academic project, developed under the open access initiative LANKESTERIANA 12(2): 93—99. 2012. THrEE NEW sHoWY BUT ENDANGErED CYRTOCHILUM sPECIEs (oNCIDIINAE: orCHIDACEAE) FroM PErU STIG DALSTRÖM1,4, GUIDO DEBURGHGRAEVE2 & SAUL RUÍZ PEREZ3 1 2304 Ringling Boulevard, unit 119, Sarasota FL 34237, USA Research Associate, Lankester Botanical Garden, University of Costa Rica, Cartago, Costa Rica and National Biodiversity Centre, Serbithang, Bhutan 2 Meersstraat 147, 1770 Liedekerke, Belgium 3 Allamanda 142, Surco, Lima 33, Peru 4 Corresponding author: [email protected] ABSTRACT. Three new Cyrtochilum species from Peru that are endangered by habitat destruction, are here described, illustrated and compared with similar species. KEY WORDS: Cyrtochilum, endangered species, Orchidaceae, Oncidiinae, new species, Peru, taxonomy The genus Cyrtochilum Kunth has gone through creeping on a bracteate rhizome, oblong ovoid, ca. quite a taxonomic turmoil during its two centuries 10 × 5 cm, distantly bifoliate (terminal leaf ca. 2 long history. The trouble has mainly been caused cm above lower leaf), surrounded basally by 7-8 by difficulties in defining the genus based on floral distichous sheaths, the uppermost foliaceous. -
Evolution Along the Crassulacean Acid Metabolism Continuum
Review CSIRO PUBLISHING www.publish.csiro.au/journals/fpb Functional Plant Biology, 2010, 37, 995–1010 Evolution along the crassulacean acid metabolism continuum Katia SilveraA, Kurt M. Neubig B, W. Mark Whitten B, Norris H. Williams B, Klaus Winter C and John C. Cushman A,D ADepartment of Biochemistry and Molecular Biology, MS200, University of Nevada, Reno, NV 89557-0200, USA. BFlorida Museum of Natural History, University of Florida, Gainesville, FL 32611-7800, USA. CSmithsonian Tropical Research Institute, PO Box 0843-03092, Balboa, Ancón, Republic of Panama. DCorresponding author. Email: [email protected] This paper is part of an ongoing series: ‘The Evolution of Plant Functions’. Abstract. Crassulacean acid metabolism (CAM) is a specialised mode of photosynthesis that improves atmospheric CO2 assimilation in water-limited terrestrial and epiphytic habitats and in CO2-limited aquatic environments. In contrast with C3 and C4 plants, CAM plants take up CO2 from the atmosphere partially or predominantly at night. CAM is taxonomically widespread among vascular plants andis present inmanysucculent species that occupy semiarid regions, as well as intropical epiphytes and in some aquatic macrophytes. This water-conserving photosynthetic pathway has evolved multiple times and is found in close to 6% of vascular plant species from at least 35 families. Although many aspects of CAM molecular biology, biochemistry and ecophysiology are well understood, relatively little is known about the evolutionary origins of CAM. This review focuses on five main topics: (1) the permutations and plasticity of CAM, (2) the requirements for CAM evolution, (3) the drivers of CAM evolution, (4) the prevalence and taxonomic distribution of CAM among vascular plants with emphasis on the Orchidaceae and (5) the molecular underpinnings of CAM evolution including circadian clock regulation of gene expression. -
The Atlanta Orchid Society Bulletin
The Atlanta Orchid Society Bulletin The Atlanta Orchid Society is affiliated with the American Orchid Society, the Orchid Digest Corporation and the Mid-America Orchid Congress Newsletter Editors: Mark Reinke & Valorie Boyer www.AtlantaOrchidSociety.org November, 2014 Volume 55: Number 11 NOVEMBER MONTHLY MEETING Monday, November 10, 2014 8:00 PM Atlanta Botanical Garden, Day Hall Mark Reinke Marble Branch Farms “Honey, I Shrunk the Or-kids!” (The History of Miniature and Compact Cattleyas) For our November meeting, outgoing president and co-owner of Marble Branch Farms will reprise a program that had its debut at the January, 2008 meeting of the Atlanta A few of the more unusual miniature and compact cattleya alliance hybrids Orchid Society and has since that have been produced by Marble Branch Farms been presented to orchid societies all over the Southeast. their newest crosses that are not yet While the structure of the available to the general public. presentation is the same, it has In This Issue been updated to keep up with Minutes of the October 2014 the latest developments in the Meeting of the Page breeding of small stature cattleya Atlanta Orchid Society type orchids, both at Marble 1, 4 Minutes from the August Branch Farms and the industry as Meeting Date: October 13, 2014, 8:00 pm a whole. The entire program is Location: Day Hall, ABG lled with beautiful 2 ATLOS Volunteer Listing Attendance: 46 people photography, all of which was taken by Mark himself. 3 Events Calendar TThe meeting, with 46 people But going beyond illustrating President’s Message present, was called to order by the the beauty and fascination of president, Mark Reinke. -
First Record of a Wild Population of Laelia Dawsonii F. Dawsonii (Orchidaceae) for the State of Jalisco, Mexico & Eduardo A
LANKESTERIANA 21(1): 11–16. 2021. doi: http://dx.doi.org/10.15517/lank.v21i1.46102 FIRST RECORD OF A WILD POPULATION OF LAELIA DAWSONII F. DAWSONII (ORCHIDACEAE) FOR THE STATE OF JALISCO, MEXICO MIGUEL J. CHÁZARO-BASÁÑEZ1, ALEXANDER JIMÉNEZ-VÁZQUEZ1,3 & EDUARDO A. PÉREZ-GARCÍA2 1Laboratorio de vida silvestre, Facultad de Biología, Universidad Veracruzana, Lomas del Estadio s/n, Xalapa, Veracruz, 91000, México. 2Departamento de Ecología y Recursos Naturales, Facultad de Ciencias, Universidad Nacional Autónoma de México, Ciudad Universitaria, Coyoacán, Ciudad de México, 04510, México. 3Author for correspondence: [email protected] ABSTRACT. The existence of a wild population of Laelia dawsonii f. dawsonii is recorded for the first time in the Mexican State of Jalisco. Laelia dawsonii has a wide geographical distribution throughout the Sierra Madre del Sur (Oaxaca, Guerrero, and Jalisco); however, the populations are very isolated, and each one has very few individuals. Until now, no wild populations of this species had been registered outside of the State of Oaxaca. The population found in Jalisco is composed of about 100 plants. Due to its horticultural importance, L. dawsonii has been frequently extracted from the field, and its Oaxacan populations have been decimated since the end of the 19th century. Currently, this species is considered endangered in Mexico. RESUMEN. Se registra por primera vez la existencia de una población silvestre de Laelia dawsonii f. dawsonii en el estado de Jalisco. Laelia dawsonii tiene una amplia distribución geográfica a lo largo de la Sierra Madre del Sur (Oaxaca, Guerrero, y Jalisco); sin embargo, sus poblaciones están muy aisladas y cada una cuenta con muy pocos individuos. -
Caucaea Colombiana Uribe-Velez, Sauleda & Szlachetko an Addition
ISSN 2325-4785 New World Orchidaceae – Nomenclatural Notes Nomenclatural Note – Issue No. 80 August 18, 2020. Caucaea colombiana Uribe-Velez, Sauleda & Szlachetko an Addition to the Flora of Colombia. Carlos Uribe-Velez1, Ruben P. Sauleda2 and Dariusz L. Szlachetko3 1Calle 115 #5-23 Bogota, Colombia. 26442 SW 107 Ct. Miami, Fl, 33173. 3Department of Plant Taxonomy and Nature Conservation, University of Gdańsk, Wita Stwosza 59, 80-308 Gdańsk, Poland. Abstract A new species of Caucaea Schltr. from Norte de Santander, Colombia is described. Kraenzlin (1922) originally included the species now included in the genus Caucaea in Oncidium sect. Cucullata Kraenzlin, typified by Oncidium cucullatum Lindl. This was the first comprehensive infrageneric classification of the genus Oncidium. Stacy (1975) suggested that the species included in this section were distinctive from Oncidium. Molecular studies (Williams et al., 2001) of some of the species included in Kraenzlin’s sect. Cucullata suggest a close relation with what was the monotypic genus Caucaea Schltr., typified by Caucaea radiata (Lindl.) Schltr. Williams et al. (2001) suggested transferring the species of the sect. Cucullata to Caucaea. This concept was rejected by Dodson and Luer (2005). However, studies of gynostemium morphology (Szlachetko & Mytnik-Ejsmont, 2009) confirm the similarity of the reproductive structures between C. radiata and species of the sect. Cucullata. In addition, subsequent genetic analysis of the Oncidiinae by Neubig et al. (2012) confirmed the molecular analysis of Williams et al. (2001) demonstrating a close phylogenetic relation between C. radiata and species of the sect. Cucullata. The analysis of Williams et al. (2001) also demonstrates a clear separation between the species of Oncidium but, a close relation between species of the sect Cucullata and taxa of the genus Cyrtochilum complex. -
Comparative Vegetative Anatomy and Systematics of Oncidiinae (Maxillarieae, Orchidaceae) William Louis Stern University of Florida
CORE Metadata, citation and similar papers at core.ac.uk Provided by The Keep Eastern Illinois University The Keep Faculty Research & Creative Activity Biological Sciences January 2006 Comparative vegetative anatomy and systematics of Oncidiinae (Maxillarieae, Orchidaceae) William Louis Stern University of Florida Barbara S. Carlsward Eastern Illinois University, [email protected] Follow this and additional works at: http://thekeep.eiu.edu/bio_fac Part of the Biology Commons Recommended Citation Stern, William Louis and Carlsward, Barbara S., "Comparative vegetative anatomy and systematics of Oncidiinae (Maxillarieae, Orchidaceae)" (2006). Faculty Research & Creative Activity. 263. http://thekeep.eiu.edu/bio_fac/263 This Article is brought to you for free and open access by the Biological Sciences at The Keep. It has been accepted for inclusion in Faculty Research & Creative Activity by an authorized administrator of The Keep. For more information, please contact [email protected]. Comparative vegetative anatomy and systematics of the Oncidiinae (Maxillarieae, Orchidaceae) WILLIAM LOUIS STERN and BARBARA S. CARLSWARD Abstract Subtribe Oncidiinae comprises a vegetatively heterogeneous assemblage of species that has persistently been incapable of organization. Anatomy was considered to be a possible means to resolve the perplexity of relationships amongst the constituent taxa. The consistent occurrence of a foliar hypodermis, homogeneous mesophyll, conical silica bodies in stegmata, and ubiquitous fibre bundles in leaves provides a matrix for linKing the taxa, as do the parenchymatous pith and O-thickened endodermal cell walls in roots. However, the strict consensus of the 40 genera studied was completely unresolved, suggesting that vegetative characters alone are insufficient to assess the relationships amongst these taxa, a conclusion also reached for the remainder of Maxillarieae. -
January 2011
Tan Bark January 2011 Toowoomba Orchid Society Inc PO Box 7710, Toowoomba Mail Centre Qld, 4352 ABN: 32 603 296 231 Our thoughts and prayers go out to anyone who was affected by the floods. To everyone in our Toowoomba and regional areas and all over Queensland and Victoria who has suffered. It has been an incredible couple of weeks for all of us. Next General Meeting Friday 28th January, 2011 Meetings: Fourth Friday of each month except September and December. Meeting commences at 7.30 pm Venue: St Pauls Hall (cnr James & Phillip Streets) Membership Fee: $15 paid annually at February AGM. Website: www.toowoombaorchidsociety.org.au Disclaimer: While every effort is made to ensure the accuracy of the content of Tan Bark, the Toowoomba Orchid Society Inc accepts no liability for the views expressed by the author/s of the published article/s or for damage to or loss of plants, from actions taken by members, as a result of articles or views expressed in Tan Bark. No part of this publication is to be reproduced without the written permission of the Tan Bark editor. President: Rick Emmerson 46 976126 Secretary: Carolyn Woolf 46 590780 Treasurer: Angela Emmerson 46 976126 Editor: [email protected] HAPPY DINERS: The January lunch at the Spotted Cow was very good. We are changing the day to the first Thursday in the month now so the Happy Dinners will be at the Westbrook Tavern on 3rd February at 11.30am, hope to see you all there. March happy diners will be a breakfast at the South Our best wishes go to Heather Toowoomba Bowls Club on Hume St commencing at 8am. -
Higher Plants Part A1
APPLICATION FOR CONSENT TO RELEASE A GMO – HIGHER PLANTS PART A1: INFORMATION REQUIRED UNDER SCHEDULE 1 OF THE GENETICALY MODIFIED ORGANISMS (DELIBERATE RELEASE) REGULATIONS 2002 PART 1 General information 1. The name and address of the applicant and the name, qualifications and experience of the scientist and of every other person who will be responsible for planning and carrying out the release of the organisms and for the supervision, monitoring and safety of the release. Applicant: Rothamsted Research, West Common, Harpenden Hertfordshire, AL5 2JQ UK 2. The title of the project. Study of aphid, predator and parasitoid behaviour in wheat producing aphid alarm pheromone PART II Information relating to the parental or recipient plant 3. The full name of the plant - (a) family name, Poaceae (b) genus, Triticum (c) species, aestivum (d) subspecies, N/A (e) cultivar/breeding line, Cadenza (f) common name. Common wheat/ bread wheat 4. Information concerning - (a) the reproduction of the plant: (i) the mode or modes of reproduction, (ii) any specific factors affecting reproduction, (iii) generation time; and (b) the sexual compatibility of the plant with other cultivated or wild plant species, including the distribution in Europe of the compatible species. ai) Reproduction is sexual leading to formation of seeds. Wheat is approximately 99% autogamous under natural field conditions; with self-fertilization normally occurring before flowers open. Wheat pollen grains are relatively heavy and any that are released from the flower remain viable for between a few minutes and a few hours. Warm, dry, windy conditions may increase cross- pollination rates on a variety to variety basis (see also 6 below).