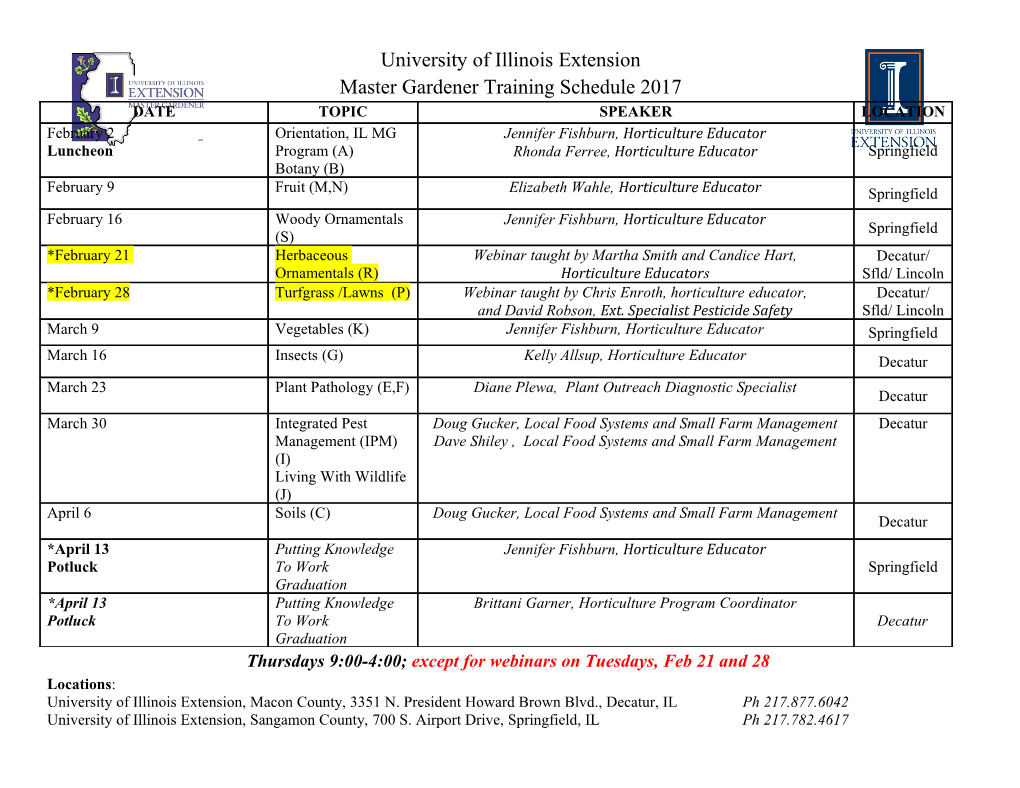
The Turgo impulse turbine; a CFD based approach to the design improvement with experimental validation David Shaun Benzon PhD Thesis SUPERVISOR: PROFESSOR GEORGE A. AGGIDIS Lancaster University in collaboration with Gilbert Gilkes & Gordon Ltd. Department of Engineering, Faculty of Science and Technology, Lancaster University, Lancaster, UK Declaration The author declares that this thesis has not been previously submitted for award of a higher degree to this or any university, and that the contents, except where otherwise stated, are the author’s own work. Signed: Date: i Abstract The use of Computational Fluid Dynamics (CFD) has become a well-established approach in the analysis and optimisation of impulse hydro turbines. Recent studies have shown that modern CFD tools combined with faster computing processors can be used to accurately simulate the operation of impulse turbine runners and injectors in timescales suitable for design optimisation studies and which correlate well with experimental results. This work has however focussed mainly on Pelton turbines and the use of CFD in the analysis and optimisation of Turgo turbines is still in its infancy, with no studies showing a complete simulation of a Turgo runner capturing the torque on the inside and outside blade surfaces and producing a reliable extrapolation of the torque and power at a given operating point. Although there have been some studies carried out in the past where injector geometries (similar for both Pelton and Turgo turbines) have been modified to improve their performance, there has been no thorough investigation of the basic injector design parameters and the influence they have on the injector performance. The aim of this research is to use modern CFD tools to develop models which aid the better understanding of Turgo impulse turbine runners and injectors and facilitate the optimisation of existing designs. CFD is used to model and optimise both the injectors and the runner of a modern commercial Turgo impulse turbine and the accuracy of the models are verified by carrying out experimental tests on the original and optimised designs. The original designs together with experience in the operation of these turbines were provided by the industrial sponsors of this research Gilbert Gilkes and Gordon Ltd. The research described in this thesis can be split into five main parts: 1. Development of a numerical model to analyses the flow through the Turgo runner using modern CFD tools combined with a series of assumptions to reduce the computational time while still retaining the accuracy of the model. Using this model to optimise the design of the Turgo runner provided by Gilkes. 2. Development of a similar numerical model for a simplified 2D injector design to facilitate a study of the impact of the basic design parameters on the performance over a range of operating conditions. Applying these optimisations to the existing Gilkes design and taking the numerical analysis further by including the full injector geometry as well as the branch pipe and guide vanes. 3. Manufacture and experimental testing of the original and optimised Turgo runners. 4. Manufacture and experimental testing of the original and optimised injector designs. ii 5. Verification of the numerical models developed in 1.) and 2.) by comparison with the experimental results. The numerical model developed in 1.) includes several simplifying assumptions in order to reduce the computational time and produce models which could solve in reasonable timescales allowing many design variations to be analysed. As the runner simulations require a transient analysis of complex multi-phase free surface flow with a rotating frame of reference they are already computationally costly and efforts have to be made to reduce this computational cost if the models are to be effective for optimisation purposes. The runner model simplifications were the exclusion of any casing interactions by not modelling the casing and the use of a 2 blade model analysing only a single blade passage in order to reduce the size of the computational domain. Several modelling assumptions were also introduced and attempts are made to quantify the effects of these assumptions through unit tests. For discretisation of the domain two mesh sizes were used, a coarse mesh which slightly under predicts the efficiency but was suitable for comparing designs and a fine mesh which gave mesh independent results. The fine mesh took over 4 times longer to solve rendering it unfeasible for optimisation purposes and it was therefore used only at key points to verify the design changes made using the coarse mesh. The analysis and optimisation of the injectors carried out in 2.) use similar CFD tools as the runner analysis however the geometry (excluding the branch pipe and guide vanes) could be simplified into a 2D axisymmetric case operating at steady state conditions. This drastically reduces the solve time and allows the use of a mesh independent model and the analysis of hundreds of designs and operating conditions. Once the optimisations had been carried out, the design changes were verified by extending the model to analyse the 3D case with a straight pipe upstream of the injector and a 3D full case including the branch pipe and guide vanes. In 3.), following the optimisation of the runner in 1.), a Finite Element Analysis (FEA) of the runner was carried out to ensure the optimised runner had sufficient strength for operation at the highest heads recommended for a runner of this size. The design was strengthened based on the results of the FEA and CFD was carried out in conjunction with these changes to ensure minimal loss in hydraulic efficiency. The manufacturing process was also researched and Design for Manufacture and Assembly (DFMA) was applied to the strengthened design identifying two optimised designs (LE4 and LE1) which will be tested before and after additional dressing of the leading edges. Both optimised runner designs were manufactured and tested at the Laboratory of Hydraulic Machines, National Technical University of Athens (NTUA). iii Following the injector analysis and optimisations in 2.), the optimised injectors were manufactured for experimental testing using both the Pelton and the Turgo test rig at NTUA in 4.). As the design changes made were not critical to the strength of the injectors there was no need to carry out a FEA. The CFD model verification in Part 5.) looks initially at the full Turgo system in order to compare the absolute difference between the numerical efficiency and the experimental efficiency of the original Turgo runner at the best efficiency point. The mechanical losses of the test rig are estimated to determine the experimental hydraulic efficiency. The numerical hydraulic efficiency is then determined by calculating the losses upstream of the injector, using standard pipe flow equations and combing these with the losses through the injector, as well as the numerical efficiency of the runner by simulating the runner using the ‘real jet’ profile produced by the full injector simulations. The results showed the numerical model to be over-predicting the efficiency by 1.26%. The numerical difference in the performance of the two injectors is then compared to the experimental difference measured during testing. This is done by importing the ‘real jet’ profiles produced by the full 3D injector simulations into the LE1 runner simulation. This allows the difference in total efficiency between the injectors combined with the runner to be compared to the experimental differences which also includes the impact of the jet on the runner performance. The comparison between the injectors is less accurate as more uncertainties are introduced when combining these models and the differences are smaller however the CFD was able to predict the improvements to within 0.4%. Finally, the numerical differences between the runner designs and the experimental differences are compared showing that the runner model is able to predict differences in hydraulic efficiency to within 0.1%. This accuracy is largely down to that fact that many of the systematic experimental and modelling errors are cancelled out when comparing only the runners. The CFD model verification has shown that although the absolute performance of the Turgo system can be modelled numerically to within a good degree of accuracy, it requires combining injector and runner models as well as estimating additional losses in the pipework which can prove time consuming. However for design comparison and optimisations the CFD models have been shown to be far more accurate suggesting that this is where these numerical models are most useful. Keywords Renewable Energy, Hydropower, Impulse turbines, Turgo turbines, Computational Fluid Dynamics, Design Optimisation, Experimental Testing iv Dedication I would like to dedicate this research to the memory of my grandfather, Philip Rudolf Botha 1923 -2000 From a young age he encouraged me to pursue a career in engineering. Here is a quote he often used, which I find a great source of inspiration. “The heights by great men reached and kept Were not attained by sudden flight, But they, while their companions slept, Were toiling upward in the night.” (From ‘The Ladder of St Augustine’ by Henry Wadsworth Longfellow) v Acknowledgements Firstly I would like to thank my supervisor Professor George Aggidis for encouraging me to study a PhD, showing continued enthusiasm in this research and for providing support and guidance. None of this would have been possible without you. My gratitude goes to Gilbert Gilkes & Gordon Ltd for sponsoring this research and supporting the experimental testing carried out in Athens. I would specifically like to thank Jonathan Martin, Alan Robinson, Dr Jo Scott and Anthony Watson for sharing their experience, providing support and giving valuable insight into this research. I would like to thank Sam Clegg, Ioannis Kassanos and Austen Wheatman for their support during the experimental testing. I would also like to thank Andy Eaton for his help in organising the presentations given at the Africa Utility Week Conference in Cape Town.
Details
-
File Typepdf
-
Upload Time-
-
Content LanguagesEnglish
-
Upload UserAnonymous/Not logged-in
-
File Pages288 Page
-
File Size-