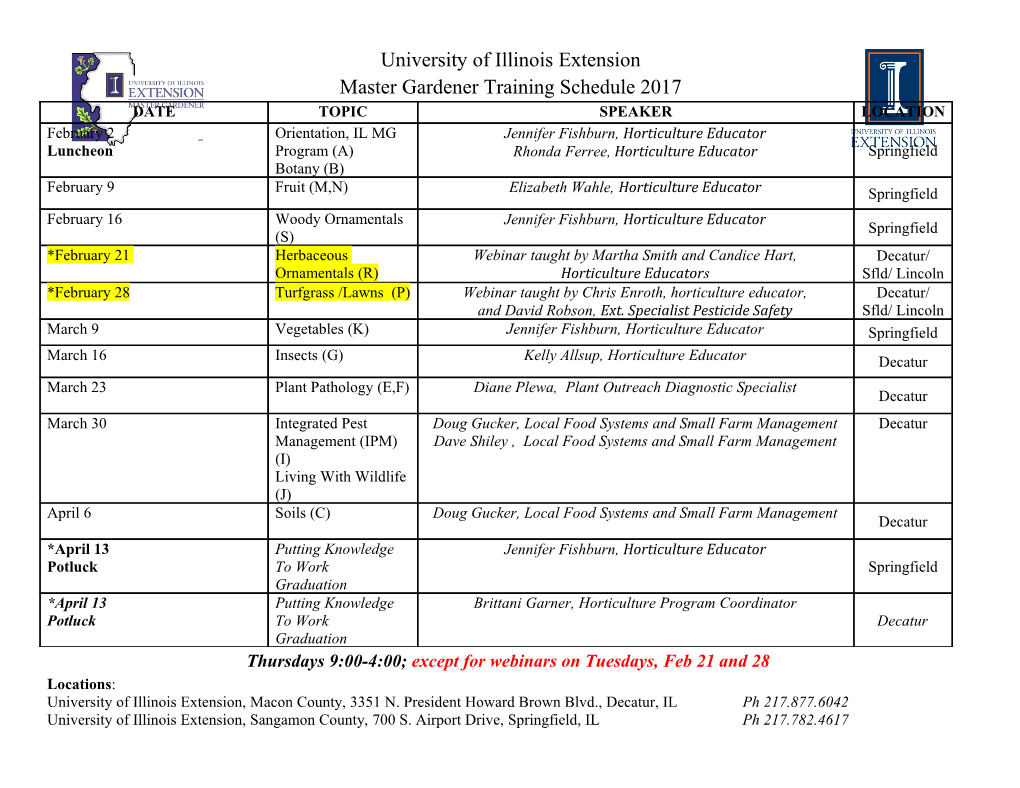
VOL. 83, NO. B9 JOURNAL OF GEOPHYSICAL RESEARCH SEPTEMBER 10, 1978 Mantle Convection and the Thermal Structure of the Plates BARRY PARSONS Departmentof Earth and PlanetarySciences, Massachusetts Institute of Technology Cambridge,Massachusetts 02139 DAN MCKENZIE Departmentof Geodesyand Geophysics,Madingley Rise, MadingleyRoad, CambridgeCB3 0EZ, England Though a simplethermal modelof plate creationmatches the observedbathymetry and heatflow with considerableaccuracy, it only doesso becausea constanttemperature is imposedas a lower boundary condition.A more realisticmodel is developedhere, basedon the idea that the thermal structureof the plate becomesunstable and leadsto the developmentof small-scaleconvection. Convective heat transport then suppliesthe heat flux neededto match the observationsrather than an artificial constanttemperature boundarycondition. The temperaturedependence of the rheologyis representedin a simplemanner. Below a given temperaturethe material is assumedto move rigidly, defining an upper mechanical boundarylayer. Beneaththis rigid layer, wherethe temperaturesare greater,the material is assumedto have a constantNewtonian viscosity.The part of the viscousregion where there are significantvertical temperaturegradients, immediately below the mechanicalboundary layer, forms a thermal boundary layer. As the plate cools,both the mechanicaland thermal boundarylayers increase in thickness.A local critical Rayleighnumber criterion is usedto testthe stabilityof the thermal boundarylayer. On this basis a convectiveinstability is predicted,its occurrencecoinciding with the breakdownof the linear depen- denceof the depthof the oceanfloor on the squareroot of age.Though the small-scaleconvection which developsfrom this instability modifies the thermal structure, the basic observationalconstraints are shown to be satisfied.The stability criterion is further testedin two differentlaboratory experiments. These experimentsalso illustrate a possibleform for the instability, with cold densematerial breaking away from the baseof the plate and being replacedby hotter material from below. 1. INTRODUCTION plied to the plate from beneath and heat lost by conduction through it. Small-scaleconvection in the upper mantle has The effectsof the cooling of the oceanicplate as it moves been suggestedas a meansof maintaining the necessaryverti- away from a midoceanridge have receiveda good deal of cal heat transport [Richter, 1973; Richter and Parsons, 1975; attention, both theoretical and observational.Forsyth [1977] McKenzie and Weiss, 1975]. In part this suggestionstemmed recentlyreviewed the different measurementsthat have been from the difficulty encounteredin numerical experimentsin made. Variations in mean heat flow, depth, and velocity of preventingconvection in boxes whose horizontal extent was surfacewaves with age all seemto reflectchange• in thermal severaltimes that of the vertical dimensionfrom breaking up structure. Parsonsand $clater [1977] have shown that varia- into many smallercells. In a model of the thermal evolutionof tionsin meandepth and'heat flow can be adequatelydescribed the plates which includesthe possibilityof small-scalecon- by a model of the type originally discussedby McKenzie vection, it is necessaryto distinguishbetween two types of [1967]. In this model a slab of constantthickness moves away boundary layers. The mechanicalboundary layer movesas a from the ridgecrest, its bottom boundarybeing maintained at rigid spherical cap and carries the magnetic anomalies. Be- constant temperature.A thicknessof 125 km for the slab is neath the mechanicalboundary layer is a thermal boundary given by a simple inversionmethod based on the analytical layer which is also cooled by heat lost upward but within propertiesof the model [Parsonsand Sclater, 1977]. which motionsnot simply relatedto the motion of the surface The phaseand group velocitiesof Rayleigh wavesfor peri- can occur. Neither boundarylayer has sharp boundaries,and ods from 20 to 200 s are found to increasewith increasingage hencethis modelis more physicallyrealistic than that usedby of the ocean floor [Kauselet al., 1974; Weidner, 1974; Leeds, McKenzie [1967]. 1975;Forsyth, 1975, 1977].These data can be invertedto give An observationthat initially seemedto support the notion the seismicvelocity structurein different age zones [Leedset of small-scaleconvection as the principal means of vertical al., 1974; Leeds, 1975; Forsyth, 1975, 1977]. Although the heat transportin the upper mantlewas the apparentapproach resolutionis limited, the overall effectof cooling in increasing of the heat flow measurementsto a constantbackground value the seismicvelocities is clear. The top of the low-velocityzone in old ocean basins.Although on closerexamination the heat is reasonablywell defined. Despite uncertaintiesabout the flow cannot in fact discriminatebetween an approachto a exactshape of the solidus[Millhollen et al., 1974;Green and constantlevel and continuedcooling, an equivalentargument Liebermann,1976], relating the sharp decrease in seismic veloci- can still be madeon the basisof the variationof depthwith age tiesto the onsetof partial meltingprovides a further constraint [Parsonsand Sclater, 1977].The meandepth of the oceanfloor on the thermal structure.Forsyth [1977] concludedthat the behavesin a very simpleway as a function of age (Figure 1). available data were consistentWith the model of a cooling Out to an age of about 70 m.y. the depth increaseslinearly as plate, 125 km thick, in a peridotiticmantle. t •/:, where t is the age of the oceanfloor. For larger agesthan Sucha model requiresa supplyof heat, the heat flux at the this the linear relationshipbreaks down, and the depth in- baseof the plate increasingfurther away from the ridge crest. creasesless rapidly, followinga simpleexponential decay to a A balance is reachedin the older regionsbetween heat sup- constantvalue. For agesyounger than 70 m.y. the form of the Copyright (D 1978 by the American GeophysicalUnion. variation can be accountedfor by a variety of thermal models. Paper number 8B0463. 4485 0148-0227/78/098 B-0463501.00 4486 PARSONSAND MCKENZIE: CONVECTION BENEATH THE OCEANIC PLATES 2000I wherethe velocityu is taken to be constant.Other termsare L NORTHPACl fib definedin Figure2. The changein depthacross the columnis !• • Meandepth and standard d.... t,on given by •- • -- Theoreticalelevahon, platemodel 3000 -' Lineart•/z relahon d(x+ dx) - d(x) = apo _ (po - pw) ßfo [T(x + dx, z) - T(x, z)] dz (2) where a is the volumetricexpansion coefficient, po the refer- 4000- encemantle density, and pwthe densityof seawaterand both (1) and (2) are correctto firstorder in (aT). The differencein internalenergy between material leaving and enteringthe col- 5000 T umn is thereforeproportional to the changein depth.Com- 6000 bining (1) and (2) resultsin the relation -(po-pw)C•, Od + q,= qo+ H(z)dz (3) 5 6 8 13 fo 7ooo I I 0 I 2 :5 4 5 6 7 8 9 I0 II 12 1:5 wheret = x/u, the ageof the oceanfloorß As d(t) andq•(t) are •/•GE (M.Y. B. P.) presumedknown, then the right-hand side of (3)is alsoknown Fig. 1. Plot of meandepth in the North Pacificversus the square as a functionof t. If q0 and H(z) can be neglected,then root of age. Numbers at the bottom of the figure denote selected Cenozoicand Mesozoicmagnetic anomalies [from Parsonsand Scla- equatingthe left-handside of (3) to zeroprovides a relation let, 1977]. betweenvariations of depthand surface heat flux. Lister [ 1977] derivedsuch a relationshipfrom the t•/•' and t -•/•' dependences_ of the two observableswhich hold for small enought. Thermal modelsof a platethat predictthe observedfunctions d(t) and One is the coolingplate model described above. Another sim- q•(t) haveidentical total heat sources given by theright-hand ply allowsthe materialto loseheat at the upperboundary sideof (3). They differonly in theway that the heatsources are without restrictingthe depth to which cooling can occur partitionedbetween q0 and H(z) and,more important, in the [Davisand Lister, 1974].A third modelallows the bottom heat transportmechanism used to supplyqo. Forsyth [1978] boundaryto migrateand assumes that it isat themelting point suggestedthat the requiredheating could be providedby ra- of the material[Parker and Oldenburg, 1973]. For sufficiently dioactive sourcesdistributed in the upper 300 km of the smallages the behavior in all thesemodels is principally deter- mantle, the vertical heat transport mechanism being con- minedby coolingfrom similar initial temperature profiles that ductive.Schubert et al. [1976] usedshear stress heating pro- are uniformwith depthright up to or almostup to the upper ducedin the large-scaleflow associatedwith the platemotion. boundary.However, for greaterages the coolinghas pene- The verticalheat transport mechanism was still predominantly trateddeep enough for theinfluence of somebottom boundary conductive.Each of the above mechanismsproduces similar conditionto be felt. Heat is suppliedso that the depthto any thermal structuresand hencecan account reasonablywell for givenisotherm increases less rapidly, and accordingly,the the observations.The problemof the stabilityof a large-scale depthof theocean floor increases less rapidly. The alternatives flow with sucha thermal structurein the upperboundary layer to the plate modelcan be modifiedto allow for an input of hasnot beenconsidered. This providesa meansof discrimina- heat from below[Crough, 1975; OMenburg, 1975], but thento ting betweenthe differentmodels, since some form of small-
Details
-
File Typepdf
-
Upload Time-
-
Content LanguagesEnglish
-
Upload UserAnonymous/Not logged-in
-
File Pages12 Page
-
File Size-