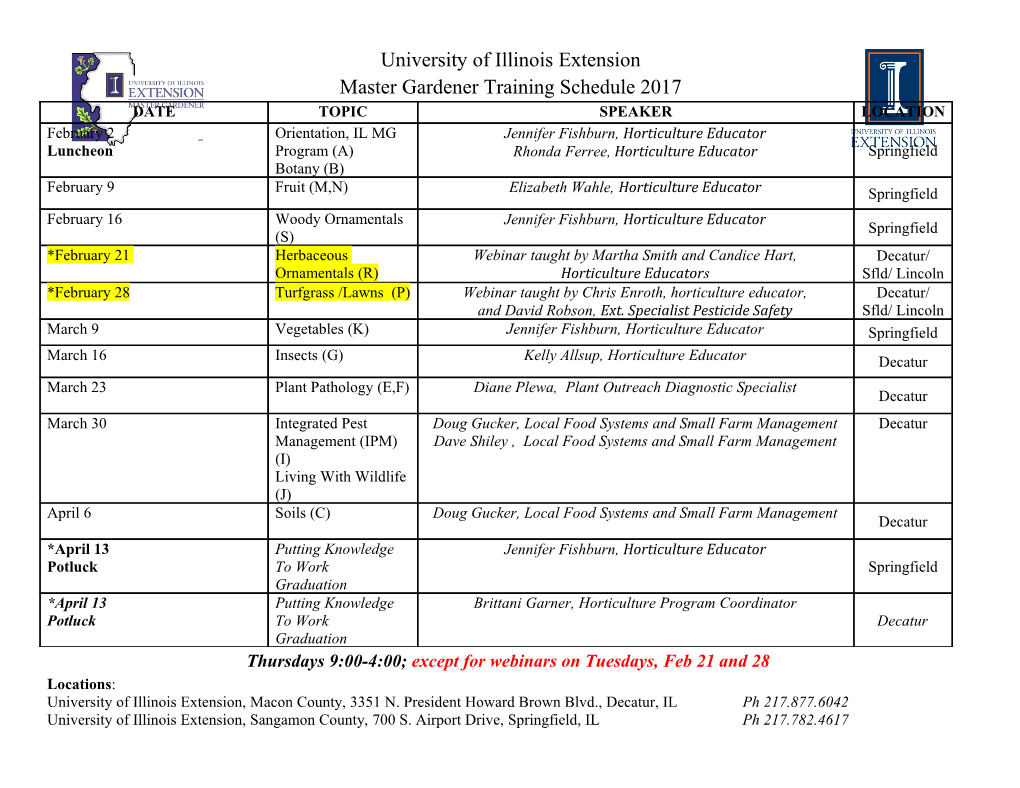
University of Groningen Engineering of sugar metabolism in Lactococcus lactis Pool, Weia Arianne IMPORTANT NOTE: You are advised to consult the publisher's version (publisher's PDF) if you wish to cite from it. Please check the document version below. Document Version Publisher's PDF, also known as Version of record Publication date: 2008 Link to publication in University of Groningen/UMCG research database Citation for published version (APA): Pool, W. A. (2008). Engineering of sugar metabolism in Lactococcus lactis. s.n. Copyright Other than for strictly personal use, it is not permitted to download or to forward/distribute the text or part of it without the consent of the author(s) and/or copyright holder(s), unless the work is under an open content license (like Creative Commons). The publication may also be distributed here under the terms of Article 25fa of the Dutch Copyright Act, indicated by the “Taverne” license. More information can be found on the University of Groningen website: https://www.rug.nl/library/open-access/self-archiving-pure/taverne- amendment. Take-down policy If you believe that this document breaches copyright please contact us providing details, and we will remove access to the work immediately and investigate your claim. Downloaded from the University of Groningen/UMCG research database (Pure): http://www.rug.nl/research/portal. For technical reasons the number of authors shown on this cover page is limited to 10 maximum. Download date: 06-10-2021 CHAPTER 3 FUNCTIONAL CHARACTERIZATION OF THREE DIFFERENT GLUCOSE UPTAKE ROUTES IN LACTOCOCCUS LACTIS Wietske A. Pool, Ana R. Neves, Helena Santos, Oscar P. Kuipers, Jan Kok. Chapter 3 52 Glucose transport and metabolism SUMMARY Molecular and physiological characteristics of lactococcal strains harbouring mutations in three different glucose uptake or dissimilation routes were determined, to gain insight into the specific roles of the two glucose-transporting phosphoenolpyruvate-dependent phosphotransfer systems (PEP:PTS) (EIIglc/man and EIIcel/glc) and the non-PTS glucose transport route(s) in Lactococcus lactis. Disruption of any of the pathways resulted in a considerable change in glucose transport. EIIcel/glc and the non-PTS route(s), had a preference for transport of the β-anomer of glucose. The main route for glucose transport was via EIIglc/man. Furthermore, a regulatory role for glucokinase (Glk) is proposed. Disruption of either glk, EIIglc/man, EIIcel/glc or both PTS systems had significant effects on overall glucose metabolism: changes in the pools of intracellular metabolites (fructose-1,6-bisphosphate (FBP), 3-phosphoglycerate (3PGA) and phosphoenolpyruvate (PEP)), in end-products formed (PEP, 3PGA, lactate, acetate, ethanol, 2,3-butanediol), and in key enzyme activities were observed. The lower lactate dehydrogenase (LDH) and pyruvate kinase (PK) activities, which might be caused by a lower FBP pool, together with transcriptional regulation, indicated by the induction of the gene encoding pyruvate formate lyase (pfl) in the glucose-PTS negative strain, resulted in the shifted fermentation pattern. This study demonstrates and specifies the roles that the three sugar uptake systems play in directing glucose uptake and subsequent metabolic processes in L. lactis. 53 Chapter 3 INTRODUCTION Lactococcus lactis, a lactic acid bacterium used in the dairy industry for the production of fermented milk products, has been studied intensely over the last decades. Its relatively simple carbon metabolism and the availability of a number of molecular cloning tools make L. lactis an attractive organism for in depth studies on sugar utilization (84, 96). In L. lactis, glucose is taken up either via a phosphoenolpyruvate-dependent phosphotransferase system (PEP:PTS), or it is imported via a non-PTS permease, and phophorylated by glucokinase (Fig. 1). The PTS is considered to be the main route for glucose metabolism (36, 148, 191). PEP:PTSs are group translocators, which import and phosphorylate sugars via a phosphoryl-transfer process. The sugar-specific enzyme II (EII) imports the sugar, while the non-sugar-specific phosphocarriers enzyme I (EI) and histidine protein (HPr) are involved in the phosphate cascade in which the phosphate moiety of PEP is transferred to the incoming sugar (42, 148, 205). Recently, we have shown that L. lactis can import glucose via two different PTS-systems, the mannose/glucose-PTS encoded by ptnABCD, and the cellobiose/glucose-PTS specified by ptcBAC (141). PTSman/glc consists of a cytosolic EIIAB protein and an integral membrane EIICD protein, the PTScel/glc is made up of a cytosolic EIIAB protein and an integral membrane EIIC protein. The glucokinase of L. lactis is an ATP-dependent protein of 33.8 kD (based on the nucleotide sequence of glk), which is able to phosphorylate intracellular glucose (195). The protein has three domains: an ATP-binding site at the N-terminus, a NagC-domain, specifying a possible transcriptional regulatory site, spanning almost the complete protein and a ROK-motif also present in certain sugar kinases and regulator proteins, in the middle of the protein. The main role of glucokinase in L. lactis is so far thought to be the prevention of accumulation of unphosphorylated glucose from the hydrolysis of disaccharides, such as lactose (195). Therefore, a deletion of glucokinase is expected to have no or at most a limited effect on the metabolism of the monosaccharide glucose in L. lactis.. The main end-product of lactococcal sugar fermentation is lactic acid (homolactic fermentation). The control of the flux through glycolysis has been studied extensively over the last decades: glycolytic intermediates have been determined using different conditions, and several glycolytic enzymes and their effectors have been described (122). 54 Glucose transport and metabolism Figure 1: Glucose Schematic Out overview of Non- glucose Membrane EIIman/glc EIIcel/glc PTS metabolism in L. In PEP lactis NZ9000. ATP Pyruvate α- And β-glucose GLK can be imported via Glucose Glucose-6P PTSman/glc (EII man/glc; ATP cel/glc ADP + Pi ptnABCD), PTS ADP + Pi (EII cel/glc; ptcBAC), or FBP by (an) as yet unidentified non-PTS glucose DHAP GAP transporter(s). The + NAD points of formation ADP + Pi NADH ATP or expenditure of GAPDH ATP and NADH are 3-PGA depicted as well as some of the enzymes involved PEP (bold). ADP + Pi PK Abbreviations: ATP NADH NAD+ glucose-6P, glucose- 6-phosphate; FBP, fructose-1,6- α-Acetolactate Pyruvate LDH Lactate ALS bisphosphate; O2 NAD+ CO DHAP, CO 2 PFL PDH NADH 2 Formate dihydroxyacetone phosphate; GAP, Diacetyl CO Acetyl-CoA 2 glyceraldehyde-3- NADH ADP + Pi 2NADH NAD+ Acetoin phosphate; 3-PGA, ATP 2NAD+ NADH 3-phosphoglycerate; NAD+ Acetate Ethanol PEP, phosphoenolpyruvat 2,3-Butanediol e; CO2, carbon dioxide; O2, oxygen; ATP, adenosine triphosphate; ADP, adenosine diphosphate; Pi, inorganic phosphate; NAD+, nicotinamide adenine nucleotide; NADH, dihydronicotinamide adenine dinucleotide; GLK, glucokinase; GAPDH, glyceraldehyde-3-phosphate dehydrogenase; PK, pyruvate kinase; LDH, lactate dehydrogenase; PFL, pyruvate formate lyase; PDH, pyruvate dehydrogenase; ALS, α-acetolactate synthase. 55 Chapter 3 Although L. lactis is generally homofermentative, under conditions of limited glucose availability or when it has to metabolize less favourable sugars, it can perform a mixed-acid fermentation in which besides lactate also acetate, ethanol, 2,3- butanediol and formate are produced (30, 67, 112).Regulation takes place at several points in the glycolytic pathway. A high level of fructose-1,6-bisphosphate (FBP) is known to activate lactate dehydrogenase (LDH) and pyruvate kinase (PK), while high levels of inorganic phosphate (Pi) together with low FBP-concentrations (when the cells are starved) inhibit PK (109, 153, 186, 191, 199). Inhibition of PK leads to accumulation of phosphoenolpyruvate (PEP) and 3-phosphoglycerate (3PGA) (152). Pyruvate metabolism is also influenced by concentrations of the glycolytic intermediates FBP, glyceraldehyde-3-phosphate (GAP), and dihydroxyacetone- phosphate (DHAP). Under anaerobic conditions LDH and pyruvate formate lyase (PFL) compete for pyruvate. During fast growth, high FBP-levels are present that activate LDH, while the high levels of DHAP and GAP under these conditions inhibit the activity of PFL (50). When a less favourable sugar is used or growth rates are lowered, less FBP, DHAP, and GAP accumulate, relieving PFL inactivation, enabling the enzyme to metabolize some of the pyruvate. Regulation of glycolysis by redox- and energy-state modulation has also been described (50). The intracellular NADH / NAD+ ratio has been suggested to control the flux through glycolysis mainly via the activity of GAP-dehydrogenase, producing NADH (50), while other studies measuring metabolites in vivo suggest a more important role for the ATP/ADP/Pi content of the cells (127, 132). In addition to regulation by glycolytic intermediates, sugar metabolism in L. lactis is also regulated at the transcriptional level via the transcriptional regulator CcpA (carbon catabolite protein A), which is involved in global metabolic control (105). Recently, L. lactis CcpA was found to intertwine regulation of carbon and nitrogen metabolism by regulating pepQ (225), a gene located tail-to-tail to ccpA (55). Besides being part of the PTS sugar phosphorylation cascade, which ultimately leads to the transfer of a phosphate-group from PEP to the incoming sugar, HPr also plays a role in regulation, since
Details
-
File Typepdf
-
Upload Time-
-
Content LanguagesEnglish
-
Upload UserAnonymous/Not logged-in
-
File Pages31 Page
-
File Size-