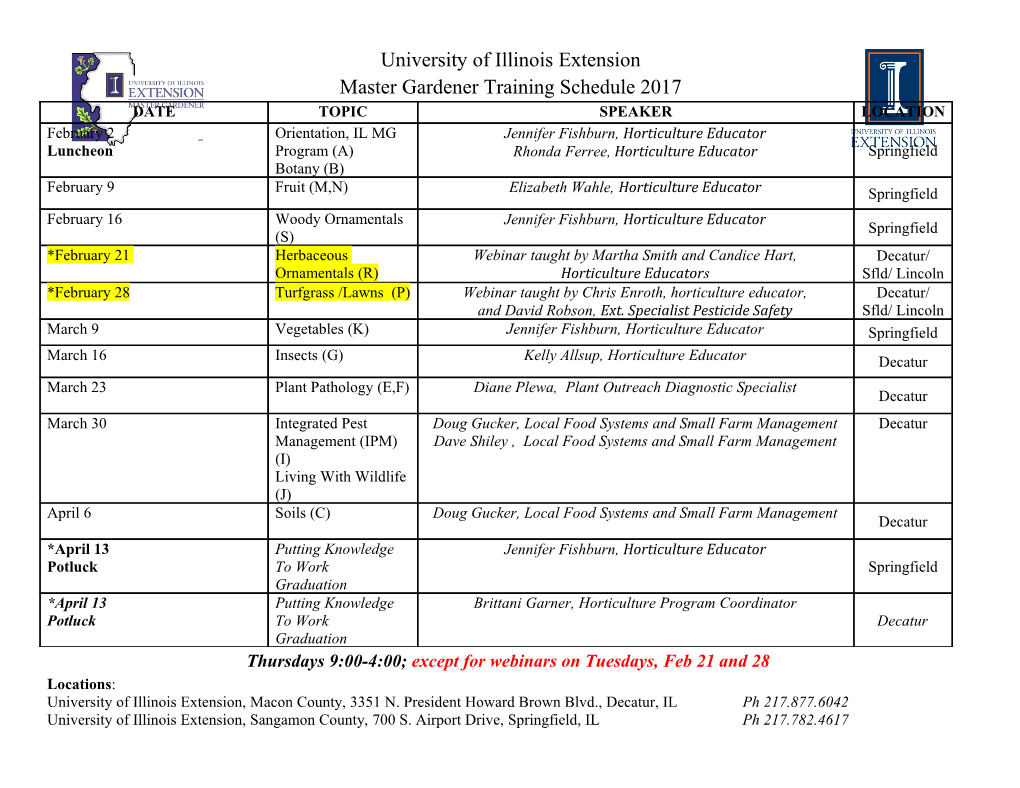
39 Mars Ice: Intermediate and Distant Past James W. Head Brown University Providence, RI [email protected] 37 Follow the Water on Mars: 1. Introduction: Current Environments and the Traditional View of Mars Climate History. 2. Recent changes in the understanding of Mars climate history: 3. Recent ice ages? The latitude-dependent layer. 4. High latitude ice reservoirs: The record of the north polar cap. 5. Mid-latitude ice deposits: Lobate debris aprons. 6. Mid-latitude ice deposits: Lineated valley fill and valley glaciers? 7. Tropical mountain glaciers: The Tharsis volcanoes. 8. Synthesis: The Amazonian: Climate History, Water, and Lessons for Life? - Heavy impact -Volcanism. - Low impact rates. bombardment. - Outflow - Tharsis volcanism continues. channels. - Valley - Early outflow channels. networks. - Oceans? - Late-stage polar caps. - “Warm/Wet” - South circumpolar - “Cold/Dry” late Mars. early Mars? deposits. The Amazonian Period of Mars History (Present to ~3 Ga) 1. Hydrological cycle: Reservoirs dominantly layered: -Atmosphere, cryosphere (surface and subsurface), groundwater. -Surface migration of volatiles is dominant climate change response. 2. Astronomical cycles are the dominant forcing function in climate change. 3. Present not typical; higher obliquity common. -Mars polar caps may be transient features. 4. More typical conditions might be: -Small to no polar caps, mid-latitude glaciation and ice sheets, tropical mountain glaciation. 5. Biological implications: -Large-scale, short term migration of microenvironments. -Local transient melting possible. -Transport mechanisms: dust/ice transport linked. -Look to Antarctic Dry Valleys for analogs. 39 Mars Ice: Intermediate and Distant Past: 1. Overview of Early Mars: Noachian and Hesperian. 2. Hesperian Mars: Outflow channels: Release, transport, and fate of ground water. 3. Hesperian Mars: Oceans on Mars?: When, where and for how long? 4. Hesperian Mars: South circumpolar ice sheets: Evidence for wet based glaciers and groundwater recharge. 5. Noachian Mars: Warm and wet? The valley networks. 6. Noachian Mars: Crater lakes and the Gusev paradox. 7. Noachian Mars: Warm and wet or cold and dry? 8. Synthesis: Where and how to look for extant and extinct life. - Heavy impact -Volcanism. - Low impact rates. bombardment. - Outflow - Tharsis volcanism continues. channels. - Valley - Early outflow channels. networks. - Oceans? - Late-stage polar caps. - “Warm/Wet” - South circumpolar - “Cold/Dry” late Mars. early Mars? deposits. 39 Mars Ice: Intermediate and Distant Past: 1. Overview of Early Mars: Noachian and Hesperian. 2. Hesperian Mars: Outflow channels: Release, transport, and fate of ground water. 3. Hesperian Mars: Oceans on Mars?: When, where and for how long? 4. Hesperian Mars: South circumpolar ice sheets: Evidence for wet based glaciers and groundwater recharge. 5. Noachian Mars: Warm and wet? The valley networks. 6. Noachian Mars: Crater lakes and the Gusev paradox. 7. Noachian Mars: Warm and wet or cold and dry? 8. Synthesis: Where and how to look for extant and extinct life. Ground Ice: Cryosphere and global aquifer system • Cryosphere is the primary reservoir for water on Mars. • Thickness of cryosphere is limited by internal heat flux. • Liquid water is stable below the cryosphere. • A global aquifer system should exist on Mars; upper boundary is bottom of cryosphere; lower boundary is depth of self compaction (~ 10 km). Outflow Channels • The most spectacular manifestations of fluvial activity on Mars. • Up to a few thousand km long. • Up to a few hundred km wide. • Up to a few km deep. • Start full-size at discrete sources. • Tributaries are rare, anastomosing pattern is common. Outflow channels: Characteristics. • Many outflow channels start at chaotic terrain. • Channels are broad, flat-floored, steep-sided valleys. • Within channels: streamline islands, terraced walls, grooves on floor. • These characteristics indicate that outflow channels were formed due to aqueous flooding events. Outflow channels: Mode of origin. • Outflow channels closely resemble terrestrial flood channels. • Flood channels are formed due to catastrophic release of water. • There are two main causes of martian floods: • Release of groundwater. • Draining of surface lakes. Outflow channels: Distribution. • Four main areas of outflow channel development: Chryse Planitia, Amazonis Planitia, Elysium-Utopia, Hellas Basin. • In Chryse, Amazonis and Hellas, outflow channels are Hesperian in age. In Elysium-Utopia, they are Amazonian. • The most important concentration of outflow channels is around Chryse. 39 Mars Ice: Intermediate and Distant Past: 1. Overview of Early Mars: Noachian and Hesperian. 2. Hesperian Mars: Outflow channels: Release, transport, and fate of ground water. 3. Hesperian Mars: Oceans on Mars?: When, where and for how long? 4. Hesperian Mars: South circumpolar ice sheets: Evidence for wet based glaciers and groundwater recharge. 5. Noachian Mars: Warm and wet? The valley networks. 6. Noachian Mars: Crater lakes and the Gusev paradox. 7. Noachian Mars: Warm and wet or cold and dry? 8. Synthesis: Where and how to look for extant and extinct life. Shorelines and Oceans. • Parker et al. mapped contacts 1 and 2 around northern lowlands. • Evidence for Contacts: – Progressive change in morphology. – Onlapping relationships between units. • Interpretation: – Contacts represented shorelines of ancient standing bodies of water, oceans. • Testing of ocean hypothesis: – Existence of oceans remained untested: 1) poorly known topography, 2) implications for shoreline elevations, water inventory. Shorelines and Oceans: MOLA data. • New MOLA data provide very important evidence to test for Mars oceans: -Elevation of contact 2 is close to an equipotential surface, -Surface below contact 2 is smoother at all scales, -Volume below contact 2 is equivalent to a global layer of water 100 m deep and lies within the range of estimates of available water on Mars. Fate of Outflow Channel Effluent Ponding in Northern Lowlands Under Current Mars Surface Conditions The Vastitas Borealis Formation: Sublimation Residue of an Ancient Ocean? 39 Mars Ice: Intermediate and Distant Past: 1. Overview of Early Mars: Noachian and Hesperian. 2. Hesperian Mars: Outflow channels: Release, transport, and fate of ground water. 3. Hesperian Mars: Oceans on Mars?: When, where and for how long? 4. Hesperian Mars: South circumpolar ice sheets: Evidence for wet based glaciers and groundwater recharge. 5. Noachian Mars: Warm and wet? The valley networks. 6. Noachian Mars: Crater lakes and the Gusev paradox. 7. Noachian Mars: Warm and wet or cold and dry? 8. Synthesis: Where and how to look for extant and extinct life. - Heavy impact -Volcanism. - Low impact rates. bombardment. - Outflow - Tharsis volcanism continues. channels. - Valley - Early outflow channels. networks. - Oceans? - Late-stage polar caps. - “Warm/Wet” - South circumpolar - “Cold/Dry” late Mars. early Mars? deposits. 39 Mars Ice: Intermediate and Distant Past: 1. Overview of Early Mars: Noachian and Hesperian. 2. Hesperian Mars: Outflow channels: Release, transport, and fate of ground water. 3. Hesperian Mars: Oceans on Mars?: When, where and for how long? 4. Hesperian Mars: South circumpolar ice sheets: Evidence for wet based glaciers and groundwater recharge. 5. Noachian Mars: Warm and wet? The valley networks. 6. Noachian Mars: Crater lakes and the Gusev paradox. 7. Noachian Mars: Warm and wet or cold and dry? 8. Synthesis: Where and how to look for extant and extinct life. Valley networks: • Rectangular or U-shaped cross- section. • Widths vary from <1 km to ~ 10 km. • Average length is ~60-70 km. • Occur preferentially in the southern hemisphere. • There are 827 valley networks on Mars. 759 valley networks are Noachian in age. • 34 are Hesperian in age, 34 are Amazonian. • Resemble river valleys on Earth, but not exactly. Valley networks: Planimetric shape and drainage density. • Planimetric shape of martian valley networks is simple. • Drainage density on Earth is about 2-30 km-1. • Drainage density on Mars is about 0.001-0.01 km-1. • Processes creating martian valley networks were far less efficient. Valley networks: Shape. • In many cases, the central valley has only a few tributaries. • Alcove-like terminations of tributaries and central valley suggest groundwater sapping. • When the density of valleys is great, surface runoff may play a role. Valley Network Origin: • Liquid water carves valley networks. • Erosion not sufficiently sustained to: – Develop large drainage basins. – Eliminate local depressions. • Water tended to pool in local depressions: – Soaks into ground. – Sublimates. • Does not keep overcome topography or keep pace. • Therefore, water occurred only sporadically. "Warm, Wet" Scenario Questioned: • Rapid scavenging of carbon dioxide. • Lack of evidence for abundant carbonates. • Lack of extensive weathering products. • Widespread evidence of olivine. • Effect of impact erosion. • Difficulties of climate models to produce rain. • Alternatives to producing water sporadically? Delivering Water Sporadically: • During periods of high obliquity: – Water driven off polar caps. – Deposited as snow at low to mid-latitudes. • Could such snowpacks then be melted by: – Top-down melting: Periodic climate warming. – Bottom-up melting: Enhanced heat flux. – Combination of both? Noachian Valley Networks: Conclusions • More liquid water on the surface in the Noachian. • Valley networks indicate overland flow in equatorial regions. • 1) Warm, wet (“rainy”) early Mars
Details
-
File Typepdf
-
Upload Time-
-
Content LanguagesEnglish
-
Upload UserAnonymous/Not logged-in
-
File Pages50 Page
-
File Size-