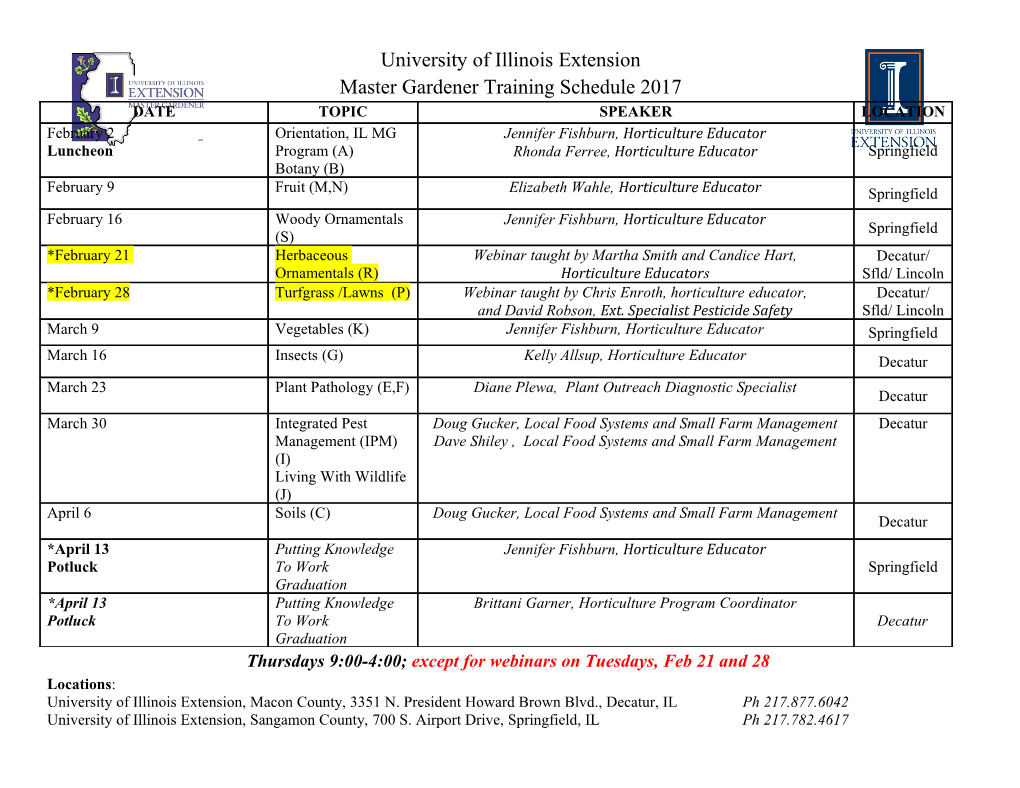
PhD. Thesis Ocean Monitoring Using L-Band Microwave Radiometry and GNSS-R Enric Valencia i Dom`enech Advised by Dr. Adriano Camps June 15, 2012 Passive Remote Sensing Laboratory Departament de Teoria del Senyal i Comunicacions Universitat Polit`ecnicade Catalunya Preface The knowledge of sea surface salinity (SSS) is a key issue to understand and monitor the Earth's water cycle. Accurate and systematic measurement of SSS was not possible until the ESA's Soil Moisture and Ocean Salinity (SMOS) mission was launched in 2009. The SMOS mission uses L-band microwave radiometry to infer SSS from measurements of the ocean's emis- sivity. However, the ocean surface emissivity is not only dependent on SSS, but also on sea surface temperature (SST), incidence angle, polarization, and sea surface roughness (i.e. sea-state). While the dependence on most of these parameters is well-known, and can be properly accounted for, the accurate estimation and correction of the sea surface roughness contribution remains a challenge. The Passive Advanced Unit (PAU) project was born in 2003 with the main objective of studying how to correct ocean L-band brightness temperature for the sea-state effect by using an emerging technology such as reflectome- try of opportunity GNSS signals (GNSS-R). GNSS-R is based on measuring the forward scattered GNSS signals so as to infer geophysical properties of the scattering surface. Particularly, the PAU project proposed to use direct observables from the reflected signal's Delay-Doppler Map (DDM) to parame- terize sea surface roughness, and link those observables to the brightness tem- perature variations induced by sea-state, without using scattering/emissivity models. In this line, prior work was performed by J.F. Marchan-Hernandez in his PhD. Thesis (UPC, Barcelona, 2009). In that work, a first version of the PAU GNSS-R receiver was developed, and first experimental results were obtained that supported the hypothesis that direct GNSS-R observables can be used to describe sea-state. This PhD. Thesis follows on that research, and steps into the use of GNSS- R observables for estimation of the sea-state contribution to the ocean L-band brightness temperature. The work presented here was undertaken between 2008 and 2012, and comprises contributions to three main fields: GNSS-R 1 hardware development, experimental results, and theoretical studies. Firstly, the PAU GNSS-R instrument was re-designed and re-implemented for im- proved sensitivity and stability. Secondly, results from ground-based and airborne experiments were obtained, that prove the hypothesis that GNSS-R observables can be used to successfully correct L-band brightness tempera- ture for the sea-state effect, resulting in an improvement in the SSS retrieval accuracy. Finally, theoretical studies to foresee the performance of the PAU concept in a future spaceborne mission were conducted, along with the de- velopment of a new technique to obtain ocean surface scattering coefficient images from measured DDMs. 2 Contents Preface1 Contents3 List of Figures9 List of Tables 21 List of Acronyms 23 1 Introduction 27 1.1 Sea surface salinity within the Earth processes . 27 1.2 Measuring sea surface salinity from space: the SMOS mission . 30 1.3 The PAU concept . 34 1.4 This PhD. Thesis . 36 2 Theoretical background 39 2.1 Microwave radiometry . 39 2.1.1 Thermal emission . 39 2.1.2 Contributions to the brightness temperature measured by a radiometer . 41 2.1.3 Brightness temperature of the ocean surface . 42 2.2 Scatterometry using GNSS signals of opportunity . 47 2.2.1 The GPS signal . 48 2.2.2 Principles of GNSS-R over the ocean . 51 I GNSS-R Hardware Development 57 3 griPAU: The GPS Reflectometer Instrument for PAU 59 3.1 Introduction . 59 3 CONTENTS 3.2 Instrument description . 62 3.2.1 Principle of operation . 62 3.2.2 Antenna and RF front-end . 64 3.2.3 Signal processor . 66 3.2.4 Clocking scheme . 72 3.2.5 Data output . 73 3.3 Instrument performance and trade-offs . 73 3.3.1 Delay estimation . 73 3.3.2 Delay-Doppler Map size and resolution . 74 3.3.3 Instrument sensitivity and stability . 77 3.4 Conclusions . 80 II Experimental Results 83 4 ALBATROSS 2009: The Advanced L-BAnd emissiviTy and Reflectivity Observations of the Sea Surface field experiment 85 4.1 Introduction . 85 4.2 The ALBATROSS field experiment . 88 4.2.1 Measurement site . 88 4.2.2 Instruments overview . 88 4.2.2.1 GPS Reflectometer Instrument for PAU (gri- PAU) ...................... 88 4.2.2.2 L-band Total Power Radiometer . 90 4.2.3 Measurement setup and ground truth data . 91 4.3 GNSS-R sea state direct descriptors . 93 4.4 GNSS-R observables relationship with the brightness temper- ature variations . 99 4.5 Experimental determination of the sea correlation time . 103 4.5.1 Methodology . 103 4.5.2 Results . 104 4 CONTENTS 4.6 Conclusions . 110 5 The CoSMOS field experiment 111 5.1 Introduction . 111 5.2 Available radiometric, GNSS-R and ground-truth data . 113 5.2.1 Radiometric data . 113 5.2.2 GNSS-R data . 115 5.2.3 Ground-truth data . 117 5.3 Brightness temperature correction using GNSS-R data . 118 5.4 Sea surface salinity retrieval . 124 5.5 Comparison with the WISE correction approach . 127 5.6 Conclusions . 129 6 Wind direction retrieval from airborne measurements 131 6.1 Introduction . 131 6.2 Observation of measured data and validation of the P2EPS simulator . 134 6.3 Modeling the DDM asymmetry . 136 6.4 Validation of the skewness angle model using real data . 143 6.5 Wind direction retrieval . 145 6.6 Conclusions . 150 7 The PARIS Interferometric Technique - Proof of Concept experiment 153 7.1 Introduction . 153 7.2 Hardware development . 157 7.2.1 Antennas . 157 7.2.2 RF front-end and calibration subsystem . 164 7.3 Study of the sea surface roughness within the PIT-PoC Ex- periment . 168 7.3.1 Experimental setup . 168 5 CONTENTS 7.3.1.1 Ground truth data . 168 7.3.1.2 L-band Radiometer . 169 7.3.1.3 GNSS-R receiver . 170 7.3.2 Measurements and results . 170 7.3.2.1 Ground-truth data . 171 7.3.2.2 Radiometric measurements . 171 7.3.2.3 GNSS-R measurements . 173 7.4 Conclusions . 179 III Towards Spaceborne Sea State Monitoring Using GNSS-R Techniques 181 8 On the use of direct GNSS-R observables for sea state mon- itoring from space 183 8.1 Introduction . 183 8.2 Simulation setup . 185 8.2.1 Considered observation geometries . 185 8.2.2 Considered Delay-Doppler Mapping conditions . 186 8.3 Comparison of the observation geometry's impact on direct GNSS-R observables . 188 8.4 Performance assessment of the normalized DDM volume . 192 8.4.1 Impact of the scenario . 192 8.4.1.1 Transmitter's flying direction effect . 192 8.4.1.2 Local incidence angle effect . 193 8.4.1.3 Receiver's flying direction and wind direction effects . 195 8.4.2 Impact of noise on the normalized DDM volume per- formance . 198 6 CONTENTS 8.4.3 Relationship with brightness temperature variations in- duced by the sea-state . 201 8.5 Conclusions . 205 9 Ocean surface imaging from DDM deconvolution 209 9.1 Introduction . 209 9.2 Theoretical background . 213 9.3 GNSS-R imaging of the ocean surface . 214 9.3.1 DDM deconvolution . 214 9.3.2 Unambiguous retrieval of the scattering coefficient dis- tribution . 214 9.4 Method evaluation . 217 9.5 Application to oil slick detection . 224 9.5.1 Oil slick modeling . 225 9.5.2 Simulation results . 226 9.6 Conclusions . 233 10 Conclusions and future research lines 235 10.1 Conclusions . 235 10.1.1 GNSS-R ocean scatterometry . 236 10.1.2 L-band brightness temperature correction for the sea- state effect using GNSS-R . 238 10.2 Future work lines . 239 Appendices 241 Appendix A GNSS-R Delay-Doppler Maps over land: Results of the GRAJO field experiment 243 A.1 Introduction . 243 A.2 Measurement setup . 245 A.3 Soil moisture retrieval . 246 A.4 Conclusions . 250 7 CONTENTS List of Publications 251 Bibliography 255 8 List of Figures 1.1 The Earth's water cycle [1]. ................... 28 1.2 Thermohaline circulation acts as a global conveyor belt that redistributes heat throughout the whole planet [2]. 29 1.3 Existing SSS measurements (scale in PSU). Gray areas repre- sent absence of data [5]. ..................... 30 1.4 Global SSS map retrieved by the SMOS Barcelona Expert Center from SMOS data (www.smos-bec.icm.csic.es). 31 1.5 SMOS observation geometry. The field of view has a hexagon- like shape of 1000 km approximately [1]. 33 1.6 Conceptual topology of an elementary PAU receiver. 35 2.1 Components of the apparent temperature Tap [22]. 42 2.2 Ocean brightness temperature as a function of SST and SSS for a flat sea surface [23]. Average salinity in open ocean is around 35 psu. 45 2.3 Sensitivities of the ∆TB term as a function of the sea state described by: a) wind speed [K/(m/s)], and b) significant wave height [K/m] [27]. ........................ 46 2.4 Radio Navigation Satellite Service band distribution after the World Radio Conference, Istambul, 8 May-2 June 2000, which discussed the allocation of the GALILEO signal spectrum. E and C bands (blue) are assigned to GALILEO, L bands (green) are for GPS, and G bands (red) are reserved for the GLONASS signals [29]. ............................ 47 2.5 a) Autocorrelation, and b) spectrum of the GPS C/A code.
Details
-
File Typepdf
-
Upload Time-
-
Content LanguagesEnglish
-
Upload UserAnonymous/Not logged-in
-
File Pages268 Page
-
File Size-