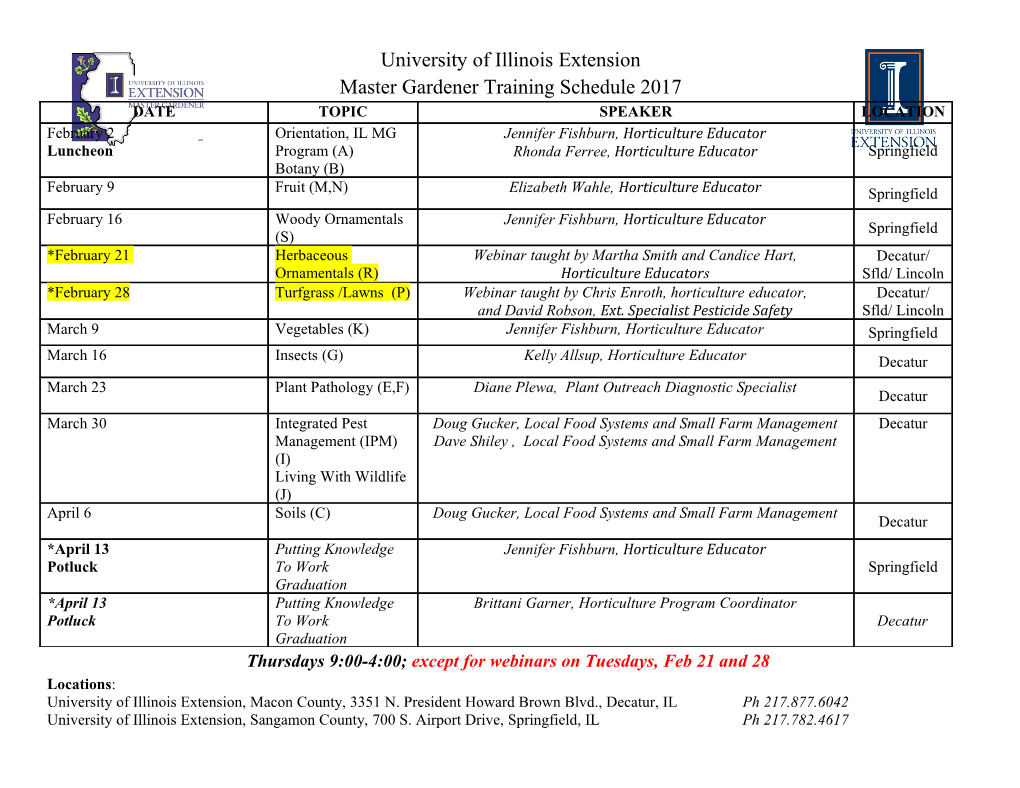
PROELECTROPHILE-NUCLEOPHILE ADDUCT FORMATION AND PERMANGANATE OXIDATION OF ALKENES: USE OF MODEL COMPOUNDS TO PROBE COMPLEX REACTION PATHWAYS By Phillip M. Flanders A dissertation submitted to Johns Hopkins University in conformity with the requirements for the degree of Doctor of Philosophy Baltimore, Maryland February, 2014 ©2014 Phillip Flanders All Rights Reserved Abstract Laboratory studies of reactions between model compounds yield valuable insights into complex reaction chemistries that would be difficult if not impossible to observe directly in environmental media. We used model compounds to investigate two types of reaction pathways: adduct formation and permanganate oxidation. First, we mimicked covalent bond forming reactions between nucleophilic contaminants and electrophilic sites within natural organic matter (NOM) or naturally occurring elec- trophilic toxicants. Initial studies modeled adduct formation at electrophilic NOM sites by reacting the model electrophiles p-benzoquinone and patulin with a suite of model nucle- ophiles. Further studies used MnO2(s, pyrolusite)-oxidized hydroquinone proelectrophiles to simulate the highly electrophilic moieties that can be generated when NOM reacts with oxidants in a sediment or soil. Overall, our model compound studies demonstrated that – added nucleophiles compete with H2O/OH to form monoadducts, which can then undergo further oxidization and addition to form di- and triadducts. Mass spectra consistent with monoadducts from reactions between nine nucleophiles and MnO2-oxidized gentisic acid were acquired by LC/MS. Separate experiments monitoring both the consumption of a model proelectrophile and a nucleophile (acetylhydroquinone and 4-ethylaniline, respectively) and II the generation of Mn from reduction of MnO2 provided indirect evidence for multiple oxidation-addition steps. ii Second, we used model compounds to study permanganate oxidation of alkenes. Compe- tition between hydrolysis and oxidation of a common intermediate leads to a distribution of products. To collect direct evidence of the influence of pH and oxidant dose on this product distribution, we selected cis-stilbenedicarboxylic acid as a model compound because it yields permanganate oxidation products amenable to LC/MS analysis. High permanganate dose favors highly oxidized products, while alkaline pH favors products from hydrolysis. This dissertation demonstrates how strategic selection of model compounds can elucidate complex environmental contaminant removal pathways by providing an internally consistent system for the study of multiple reaction conditions and competitive reactions. Advisor: Alan T. Stone Readers: A. Lynn Roberts and Steven Rokita iii Acknowledgments I owe thanks and gratitude to many people who’ve helped me and been there for me during graduate school. First I thank my parents, Jeff and Karen Flanders, for their love and support throughout these many years of education. They have always offered inspiration, guidance, and assistance whenever I needed it–whether I knew I needed it or not. I’d like to thank my roommates who’ve lived with me during graduate school: John Sivey, Umut Aypar, Andrew Clayborne and Jennifer Ronald. They put up with my quirks over the years and were understanding front lines to the day-to-day stress of graduate school. I thank my advisor, Prof. Alan Stone, for all of his guidance, patience, and detailed feedback. He has been an invaluable resource and imaginative thinker throughout this entire process. I thank other professors at Johns Hopkins who have influenced me over the past few years: Lynn Roberts, Bill Ball, Ed Bouwer, Kai Loon Chen, and Dimitri Sverjensky. I thank my DQE committee: Alan Stone, Lynn Roberts, Ed Bouwer, and Kai Loon Chen. I thank my GBO committee: Alan Stone, Lynn Roberts, Gerry Meyer, Caren Meyers, and Gary Posner. Lastly, I thank my defense committee: Alan Stone, Lynn Roberts, and Steven Rokita. iv I thank Penney Miller, my research advisor during my undergraduate years at Rose- Hulman Institute of Technology. She piqued my interest in environmental chemistry. Her mentorship provided me with a set of skills that have been invaluable in my doctoral research. She gave me real appreciation for HPLC and LC/MS. She built the foundation of my scientific writing, presentation, and experimental planning skills. I thank Chris Higgins for teaching me how to operate the LC/MS, which I used exten- sively in my research. He also has been a continuing source of help and inspiration. I thank Keith Ritchie for all of his time and effort making sure that the instruments and tools that I use were kept in working order. I’m also grateful for his help with the lab for the Experimental Methods course. I thank the department’s front office staff for all of their help making this dissertation happen: Joe, Joyce, Christine, Rob, Rok, Adena, Denise, and the student workers. I thank the other members of Prof. Stone’s research group during my time at Johns Hopkins: Zhi Shi, Nathan Boland, Grace Stokes, and Xiaomeng Xia. I also thank the other doctoral students who worked along with me in the labs: John Sivey, Kevin Bisceglia, Katie Onesios, Jessica Lawson, Amar Wadhawan, Andrew Graham, Pavlo Bohutskyi, Jin Yang, An Huynh, Peng Yi, Yaqi You, Shao-Yiu Hsu, and Stephanie Lau. The discussions, collaborations, and friendships I’ve had with all of you were an invaluable part of these years of my life. Lastly, I need to thank the friends I have made in Baltimore for making sure that–when I have some free time–I always enjoyed myself! You guys are the best! I would also like to thank the Dissertation Support Group for your advice and inspiration. Thanks to everyone who has been a part of my life these past six years! v This dissertation is dedicated to the army of people who believed in me and made it possible. vi Contents Abstract ii Acknowledgements iv List of Tables xiii List of Figures xix List of Symbols xx 1 Introduction 1 1.1 Motivation1 1.2 Oxidation States of Manganese6 1.3 Research Objectives and Thesis Outline8 2 Aqueous Addition Reactions of Patulin and p-Benzoquinone: Competition Be- tween Added Nucleophiles, Water, and Buffers 23 2.1 Introduction 23 2.2 Materials and Methods 28 2.2.1 Chemicals 29 2.2.2 Experimental Design 29 vii 2.2.3 Instrumentation and Analysis 30 2.3 Results and Discussion 33 2.3.1 p-Benzoquinone 33 2.3.2 Patulin 35 2.3.3 Comparison of Electrophilicity: p-Benzoquinone and Patulin 35 2.3.4 Assessing Nucleophilicity: Aminopyralid, Chloramben, and Clo- pyralid 37 2.4 Conclusions 38 2.5 Supporting Information 54 2.5.1 Patulin Michael Addition Mechanism Proposed by Fliege and Metzler 54 2.5.2 p-Benzoquinone and Reactions with Buffer Compounds 57 2.5.3 Patulin and Reactions with Buffer Compounds 61 3 Highly Reactive p-Benzoquinone Electrophiles Generated by the Oxidation of Substituted Hydroquinones Using MnO2(s, pyrolusite) as an Oxidant 78 3.1 Introduction 78 3.2 Materials and Methods 87 3.2.1 Chemicals 87 3.2.2 Experimental Design 88 3.2.3 Instrumentation and Analysis 89 3.3 Results and Discussion 91 3.3.1 Proelectrophile Experiments with Acetylhydroquinone, 4-Ethyl- aniline, and MnO2 91 3.3.2 Influence of Proelectrophile and Nucleophile Structure 96 3.3.3 LC/MS Evidence of Monoadducts 99 viii 3.4 Conclusions 101 3.5 Supporting Information 123 3.6 Application of the Langmuir-Hinshelwood-Hougan-Watson kinetic model 123 4 Permanganate Oxidation of Alkenes: Studying Product Distribution Using cis- Stilbenedicarboxylic Acid as a Convenient Model Compound 144 4.1 Introduction 144 4.2 Materials and Methods 152 4.2.1 Chemicals 153 4.2.2 Reaction Medium and Experimental Design 154 4.2.3 Time Course Experiments and Kinetic Analysis 155 4.2.4 Reaction Quench Experiments and Oxidizing Equivalents 157 4.2.5 Instrumental Analysis 161 4.3 Results 162 4.3.1 Time Course Experiments 162 4.3.2 Reaction Quench Experiments and Oxidizing Equivalents 164 4.4 Discussion 166 4.5 Conclusions 170 4.6 Supporting Information 190 4.6.1 Redox Reactions for Permanganate Oxidation of an Alkene 190 4.6.2 3-Cyclopentene-1-carboxylic acid 194 5 Conclusion 208 5.1 Summary of Findings 208 5.1.1 Nucleophilic Addition Reactions 208 5.1.2 Permanganate oxidation of Alkenes 211 ix 5.2 Future Research 212 5.2.1 Nucleophilic Addition and the Proelectrophile Pathway 212 5.2.2 Oxidation by Permanganate 214 Vita 216 x List of Tables Table 1.1. Reduction half-reactions and standard reduction potentials for en- 20 vironmentally and water/wastewater treatment relevant manganese – species. For MnO4 the relevant pH range is given. Table 2.1. Second-order rate constants determined for reactions of added nu- 45 cleophiles with p-benzoquinone and patulin Michael acceptor. Rate constants (kNu− ) corrected to reflect the deprotonated form of the nucleophile (Equation 2.4). Half-life calculated for 1 mM of the deprotonated form of the nucleophile. Table 2.2. Hammett reaction constants (r) for para-substituted anilines deter- 46 mined in this study and available in the literature. Table S2.1. Pseudo-first-order rate constants observed for p-benzoquinone loss 63 in the presence of nucleophiles (kobs). Second order rate constants (k) determined by normalizing kobs by concentration of deprotonated nucleophile (Equation 2.4). Error shown determined from 95% confi- dence intervals for the slope of the best-fit line. Conditions: 5 mM MOPS and 10 mM NaCl. pKa values from Table S2.3. Hammett σ constants (7) shown for aromatic amines. Table S2.2. Pseudo-first-order rate constants observed for patulin loss in the pres- 64 ence of nucleophiles (kobs). Second order rate constants (k) deter- mined by normalizing kobs by concentration of deprotonated nucle- ophile (Equation 2.4). Error shown determined from 95% confidence intervals for the slope of the best-fit line.
Details
-
File Typepdf
-
Upload Time-
-
Content LanguagesEnglish
-
Upload UserAnonymous/Not logged-in
-
File Pages238 Page
-
File Size-