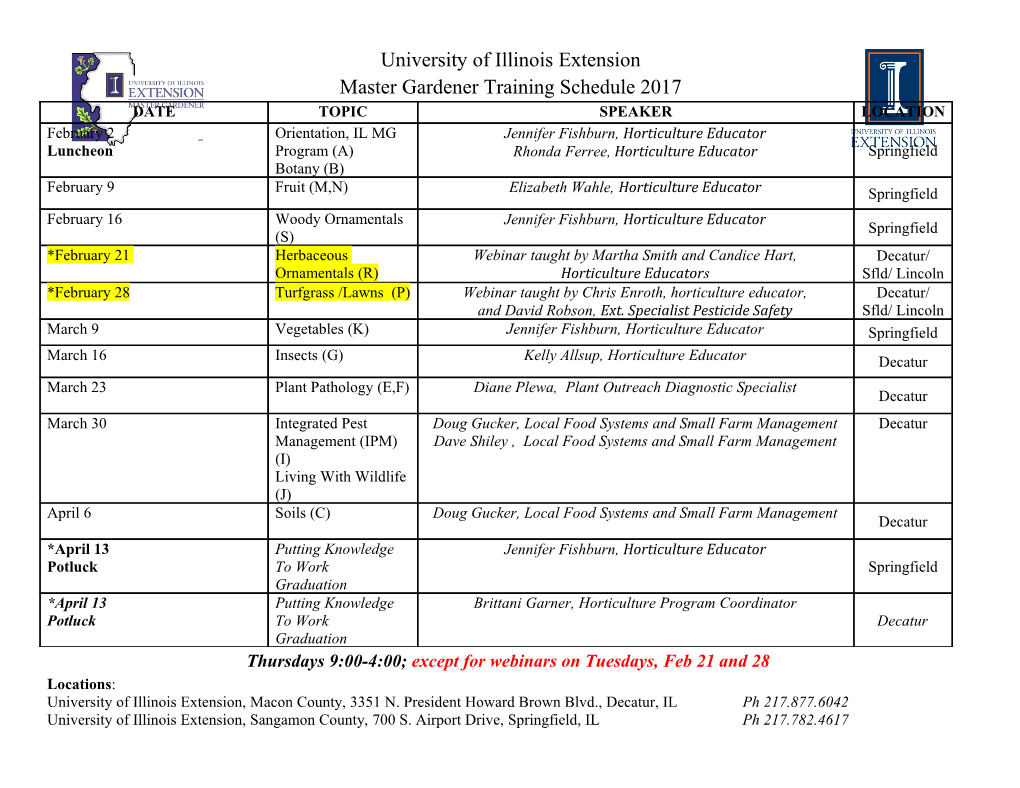
Pathways Towards Habitable Planets ASP Conference Series, Vol. 430, 2010 Vincent Coud´e du Foresto, Dawn M. Gelino, and Ignasi Ribas, eds. How to Find a Habitable Planet J. F. Kasting Department of Geosciences, The Pennsylvania State University, University Park, PA, USA Abstract. Over 400 extrasolar planets have now been discovered by ground- based methods, especially the radial velocity (RV) method. Most of these planets are much bigger than Earth, and only a handful of them are rocky planets that could conceivably harbor life. Within the next few years, we may be able to identify Earth-sized planets within the habitable zones around M stars by looking for transits, and RV techniques may even enable us to find Earths around more Sun-like (F-G-K) stars. To characterize these planets, though, we are likely to need new space-based telescopes that operate either in the visible/near-infrared or in the thermal-IR. Some conceptions of what these telescopes might look like and what we might learn from them are discussed. 1. Introduction To date (December 2009), some 405 extrasolar planets have been reported (http://exoplanet.eu/), with most of them found around nearby stars us- ing the radial velocity (RV) method. That is the good news for planet hunters and astrobiologists. The bad news is that nearly all of these planets are thought to be gas or ice giants that lack solid or liquid surfaces on which life might origi- nate and evolve. Although Carl Sagan once suggested that bubble-like creatures might hover in Jupiter’s clouds, originating life in such an unstable environment would be difficult, if not impossible. Rocky planets like Earth are much more likely abodes for life. A second requirement for detectable life is that it must be able to modify a planet’s atmosphere in ways that might be observed remotely. Earth provides an example of such a planet: Organisms, specifically photosynthetic algae, plants, and cyanobacteria, have created an atmosphere rich in O2 that is very differ- ent from the predominantly CO2 atmospheres on neighboring Mars and Venus. What allows this to happen on Earth is the presence of abundant liquid water on its surface, which provides a home for photosynthetic organisms. Liquid water is also an essential requirement for life as we know it. Clever biochemists have speculated that life might develop in other types of fluids, e.g., liquid CH4 (NRC 2007). Even if this is possible, though, such life would be so different from us that we might not be able to recognize its existence from afar. For this reason, we henceforth limit our discussion to the conventional circumstellar habitable zone (HZ), the region around a star within which a planet can maintain liquid water on its surface. Although this might seem overly restrictive, planets falling into this category may exist around many, or even most, stars. So, making 3 4 Kasting this assumption should not seriously impede our search for life elsewhere in the Galaxy. 2. Habitable Zones around Stars The concept of the circumstellar HZ was introduced by Shapley (1953), Strughold (1953), and Huang (1959). Hart (1978, 1979) extended this idea by defining the continuously habitable zone (or CHZ) as the region that remains habitable for some finite period of time as the star evolves and increases in luminosity. Hart attempted to estimate the width of both the HZ and the CHZ around the Sun and other stars. He did so by performing time-dependent numerical simulations of Earth-like planets at different orbital distances around their parent stars. Hart’s conclusions were extremely pessimistic. He found that the 4.6-Gyr CHZ around the Sun was itself rather narrow, 0.95-1.01 AU, and that CHZs around other main sequence stars were either narrower or completely nonexistent. If this conclusion was correct, Earth might be the only habitable planet in the entire Galaxy. Theories of the habitable zone evolved, partly as a consequence of studies of the so-called “faint young Sun” problem on Earth. The Sun was roughly 30 percent less luminous when the Solar System formed 4.6 billion years (b.y.) ago (Gough 1981); yet Earth has had life since ∼3.5 b.y. ago and liquid water since 4.4 b.y. ago (Valley et al. 2002). At least part of the solution to this problem is that early Earth probably had a much larger atmospheric greenhouse effect, caused by the presence of high concentrations of CO2 (Walker et al. 1981) and perhaps CH4, as well (Kiehl & Dickinson 1987; Haqq-Misra et al. 2008). CO2 concentrations would have been enhanced because of a strong negative feedback in the carbonate-silicate cycle, which controls atmospheric CO2 concentrations over long time scales. As Earth’s surface cools, silicate weathering slows down, thereby reducing the rate at which CO2 can be sequestered into carbonate rocks. Steady state can be achieved only when liquid water is present, thereby ensuring that early Earth remained within the liquid water regime. This same feedback implies that if Earth had formed further from the Sun, it should have developed a dense CO2 atmosphere that would have kept its surface warm. Thus, the outer edge of the habitable zone should be farther out than Hart calculated, probably somewhere around 1.7–2.0 AU (Kasting et al. 1993; Forget & Pierrehumbert 1997). Hence, the HZ should be relatively wide, and the 4.6-Gyr CHZ around the Sun should extend from ∼0.95 AU to ∼1.4 AU. If terrestrial planets around other stars exist, and if they are distributed in orbital distance as they are in our own Solar System, the chances of finding another habitable planet appear to be pretty good. 3. The Gliese 581 System Although none of the exoplanets discovered so far is thought to be habitable, two candidates have come extremely close. Both of them orbit the star Gliese 581. Gliese 581 is an M3V star with 0.31 times the mass of the Sun and 0.0135 times its luminosity. It has 4 known planets, b-e, whose properties are listed in Table 1. These planets were all discovered using the RV method (Udry et al. How to Find a Habitable Planet 5 Table 1. The Gliese 581 system Planet Mass (M⊕) Semi-major axis (AU) b 30.4 ≥ M ≥ 15.65 0.04 c 10.4 ≥ M ≥ 5.36 0.07 d 13.8 ≥ M ≥ 7.09 0.22 e 3.1 ≥ M ≥ 1.94 0.03 2007), and so the lower limits on planetary mass (in Earth masses) are derived in the usual manner. The upper mass limits are derived from considerations of orbital stability (Mayor et al. 2009). The two planets that are closest to being within the habitable zone are Gliese 581c and d. Planet c is most likely rocky, given that its mass is between 5 and 10 Earth masses. But the flux incident on planet c is about 30 percent greater than that hitting Venus today (von Bloh et al. 2007; Selsis et al. 2007), so planet c is unlikely to be habitable. Planet d is a better candidate, although it may or may not be rocky, as its upper mass limit is close to that of Uranus (about 14 Earth masses). Its estimated semi-major axis, 0.22 AU, has come down somewhat since it was first reported; hence, the stellar flux available at the planet’s orbit has increased to about 28 percent of that hitting the Earth (1365 W/m2). This is slightly higher than the “maximum greenhouse” limit for a slightly bluer M0 star (Kasting et al. 1993, Table III); hence, it could very well be habitable if it is indeed a rocky planet. 4. Searching for Habitable Planets by using Transits The discovery of the Gliese 581 system has demonstrated that it should already be possible to search for potentially habitable planets around M stars. One may be able to do this using the RV technique, particularly if that technique can be extended to the near-IR, where M stars are at their brightest. But the most promising technique is to look for transits (Deming et al. 2009). Finding potentially habitable planets around M stars is much easier than finding them around F-G-K stars because the stars themselves are smaller, allowing a tran- siting Earth-sized planet to produce a strong signal, and because the HZ is close to the star, increasing the probability that a transit will occur. (The transit probability is equal to the radius of the star divided by the radius of the planet’s orbit.) The odds of seeing Earth around the Sun are only 1 in 200. For a late M star, the HZ is at ∼0.03 AU and the stellar diameter is about 0.1 times that of the Sun; hence, the transit probability increases to about 1 in 60. If transiting M-star planets can be located within the next few years, it may be possible to study their atmospheres spectroscopically with the James Webb Space Telecope (JWST), scheduled for launch in 2014. The idea would be to employ secondary eclipse spectroscopy, as has been done already for hot Jupiter-type exoplanets using the Spitzer Space Telescope. In this technique, one measures the infrared emission from the combined star plus planet while the planet is visible, then subtracts the spectrum obtained when the planet is behind 6 Kasting the star. Whether or not this can yield useful information about terrestrial type planets around M stars remains to be seen, but it is an intriguing possibility.
Details
-
File Typepdf
-
Upload Time-
-
Content LanguagesEnglish
-
Upload UserAnonymous/Not logged-in
-
File Pages10 Page
-
File Size-