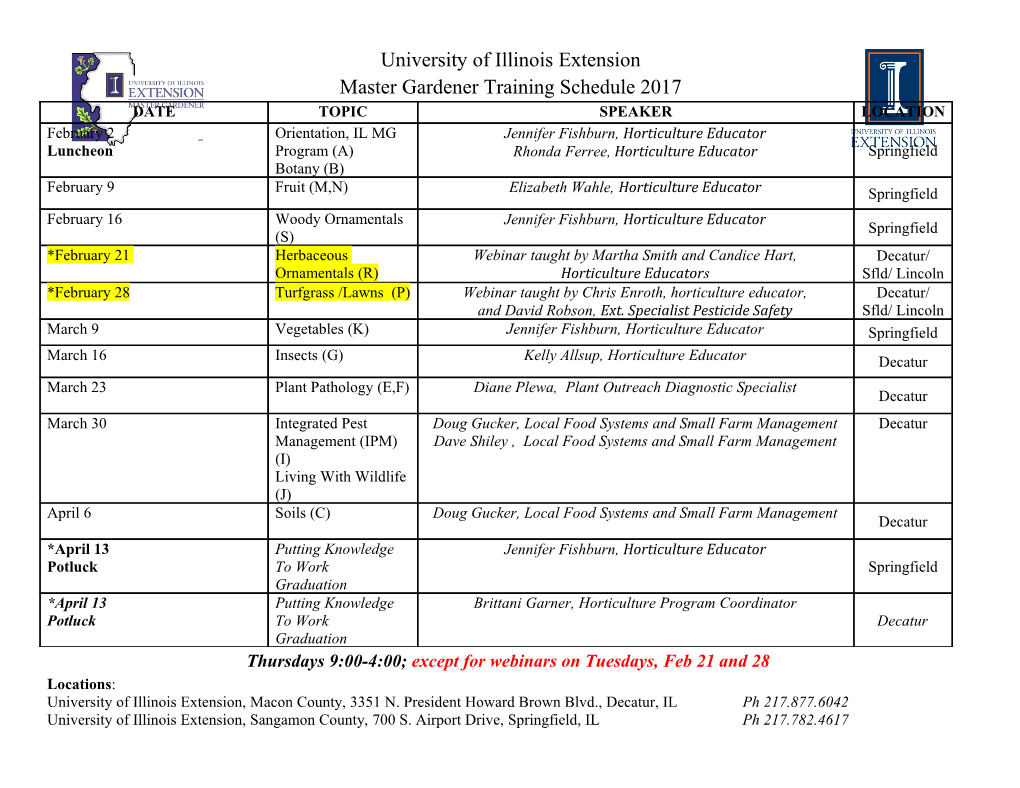
6.05 Heat Flow and Thermal Structure of the Lithosphere C. Jaupart, Institut de Physique du Globe de Paris, Paris, France J.-C. Mareschal, GEOTOP-UQAM-McGill, Montreal, QC, Canada ª 2007 Elsevier B.V. All rights reserved. 6.05.1 Introduction 218 6.05.2 Surface Heat Flux and Heat Transport in the Earth 219 6.05.2.1 Distribution of Heat Flux: Large-Scale Overview 219 6.05.2.2 Mechanisms of Heat Transport 220 6.05.2.3 Thermal Boundary Layer Structure 220 6.05.2.4 Basal Boundary Conditions 221 6.05.2.5 Controls on Lithosphere Thickness 222 6.05.2.6 The Thermal Lithosphere as Opposed to the Seismically Defined Lithosphere 222 6.05.2.7 Summary: Different Approaches to Thermal Studies of the Lithosphere 223 6.05.3 Oceanic Heat Flux, Topography and Cooling Models 223 6.05.3.1 Data 223 6.05.3.2 Hydrothermal Circulation 223 6.05.3.3 Heat Flux Measurements Near Rift Zones 224 6.05.3.4 Cooling Half-Space Model 224 6.05.3.5 Old Ocean Basins: Flattening of the Heat Flow versus Age Curve 226 6.05.3.6 Modified Thermal Model for the Oceanic Lithosphere 226 6.05.3.7 Variations of Lithospheric Thermal Structure due to Factors Other Than Age 228 6.05.3.7.1 Large-scale variations of mantle temperature 228 6.05.3.7.2 Hot spots 229 6.05.3.8 Summary 229 6.05.4 Continental Lithosphere in Steady State 229 6.05.4.1 Vertical Temperature Distribution 229 6.05.4.2 Crustal Heat Production 230 6.05.4.3 Mantle Heat Flux 233 6.05.4.4 Regional Variations of Heat Flow and Lithospheric Temperatures 234 6.05.4.5 Variations of Crustal Thickness 236 6.05.4.6 Summary 238 6.05.5 Continental Lithosphere in Transient Thermal Conditions 238 6.05.5.1 General Features 238 6.05.5.2 Compressional Orogens 238 6.05.5.3 Rifts and Zones of Extension 239 6.05.5.4 Thermal Relaxation of Thick Continental Lithosphere 239 6.05.5.4.1 Sedimentary basins 239 6.05.5.4.2 Tectonic and magmatic perturbations 240 6.05.5.5 Long-Term Transients 240 6.05.5.5.1 Archean conditions 240 6.05.5.5.2 Secular cooling in the lithosphere 241 6.05.6 Other Geophysical Constraints on the Thermal Regime of the Continental Lithosphere 242 6.05.6.1 Constraints from Seismology 242 6.05.6.2 Seismicity, Elastic Thickness, and Thermal Regime of the Lithosphere 242 6.05.6.3 Depth to the Curie Isotherms 244 6.05.6.4 Thermal Isostasy 244 6.05.7 Conclusions 244 References 246 217 218 Heat Flow and Thermal Structure of the Lithosphere 6.05.1 Introduction the oceans remain poorly sampled. Two methods have been used to circumvent this data gap. One Over the past 200 years, the cooling of the Earth has has been to search for a simple control variable on been the object of many studies (Fourier, 1820; lithospheric temperatures, such as age. Another has Thomson, 1864; Strutt, 1906; Holmes, 1915; been to develop increasingly precise and detailed McDonald, 1959; Urey, 1964; Birch, 1965). Since the seismic velocity profiles and to invert them for paper of Kelvin (Thomson, 1864), the estimate of the temperature. average heat flux has changed by a factor of less than 2, To set the stage for this chapter, we recapitulate but our understanding of this value and its implication briefly the advances made in one generation, as mea- for the Earth’s thermal structure has been turned sured against a comprehensive review paper (Sclater upside down several times. Kelvin obtained the solu- et al., 1980). When that review was published, the tion of the heat equation for the conductive cooling of oceanic heat flow problem was essentially solved. a half-space, initially at constant temperature, after a Since then, the model has passed a series of critical tests. For young sea floor, this has entailed docu- sudden dropp inffiffiffiffiffiffiffiffiffi surface temperature. Because heat flux varies as 1= time, Kelvin used the present heat flux to menting and understanding hydrothermal determine the age of the Earth. The Kelvin model is circulation through fractured oceanic crust buried not correct for two reasons. One is that the Earth is not by variable thickness of low-permeability sediments cooling by conduction only; convective motions are and determination of small-scale heat flux variations. driven by temperature differences in the mantle. For old sea floor, this has involved precise measure- Another reason is that Kelvin ignored internal heat ments of heat flux through areas with flat topography unaffected by sedimentary perturbations (slumping, sources; the amount of heat generated by the radio- turbidity currents) and deep oceanic currents. On active decay of U, Th, and K in silicate rocks accounts continents, progress has been made thanks to sys- for a large fraction of the heat flow. This was first tematic measurements of heat flux and heat pointed out by Strutt (1906) who estimated from production in old cratons and determination of heat-production measurements that crustal thickness (P, T ) conditions in the lithospheric mantle through could not exceed 60 km. Following Bullard (1939, xenolith studies. Additionally, subsidence studies in a 1954) and Revelle and Maxwell (1952), systematic large number of sedimentary basins have documen- heat flow measurements on land and at sea were ted the transient response of the continental undertaken which showed that radiogenic heat pro- lithosphere to thermal perturbations. For both con- duction is not uniformly distributed. It is worth noting tinents and oceans, detailed seismic velocity profiles that in the 1950s, when Bullard undertook oceanic have been interpreted in terms of the thermal struc- heat flux measurements, he expected heat flux to be ture of the lithosphere and mantle. Complementary lower in the oceans than in the continents, because the information has come from many disciplines, leading oceanic crust is thin and poor in radioelements. to a very large data set and to a sound understanding The concept of lithosphere, as seen from the ther- of key issues. mal standpoint, and estimates for its thickness have Here, we review these advances focusing on two also evolved. The heat flux data do not fit the age issues. One is to assess the reliability of age as a variation predicted by the half-space conductive control variable on heat flow and lithospheric ther- cooling model. In the oceans, this is because heat is mal structure. In continents, variations of crustal heat brought to the base of the lithosphere, probably production are large in both the horizontal and ver- through small-scale convection. In the continents, tical directions and prevent the calculation of a single variations of heat flux with age are obscured by typical ‘geotherm’ for all provinces of the same age. large changes of radiogenic heat production in the In oceans, age is the main control variable but small crust. variations remain due to lateral variations of mantle How continents and oceans deform and are temperature. The other issue is the control on litho- affected by magmatism depends largely on their ther- spheric thickness and the mechanism of heat mal structures. Thus, current research on geological transport at the base of the lithosphere – in other and geodynamical processes requires accurate words, the bottom boundary condition to be used in knowledge of temperatures in specific regions. thermal models. In steady state, this boundary con- Despite many decades of measurements, many geo- dition is of small concern because the thermal logical provinces on the continents and large parts of structure can be determined by downward Heat Flow and Thermal Structure of the Lithosphere 219 continuation of surface measurements. It is a major flow map of North America (Figure 1) (Blackwell factor, however, if the thermal regime is transient. and Richards, 2004) illustrates the differences We have not aimed this chapter at heat flow spe- between continental and oceanic heat flow. Except cialists and pursue two different goals. One is to show in active regions (Basin and Range, Yellowstone, Rio how to use heat flux data, which involves an analysis of Grande Rift, etc.), heat flux is low throughout the available measurements and their uncertainties as well North American continent and lowest in the as a discussion of missing information. Our other goal Canadian Shield. In contrast, heat flux is higher in is to illustrate the controls on the different thermal the oceans than in the continents, and higher on the regimes that are observed and to describe the key steps western than on the eastern margin. In addition to in data interpretation. This will be achieved with these large-scale differences, many other features simple physical arguments instead of complicated need detailed interpretation. We shall see that one numerical calculations. In the following, for simplicity, cannot apply the same physical frame-work and the data and measurements refer only to heat flow studies same interpretation methods to oceans and conti- unless otherwise specified. nents. From a global perspective, the oceanic lithosphere is in a transient thermal state over most of its short residence time at the Earth’s surface, 6.05.2 Surface Heat Flux and Heat contrary to continents which are mostly in, or close Transport in the Earth to, thermal steady state. Oceanic heat flux follows a decreasing trend as a function of age which parallels 6.05.2.1 Distribution of Heat Flux: Large- that of elevation (or bathymetry). The continental Scale Overview lithosphere has experienced a longer evolution and There are more than 20 000 heat flux measurements is characterized by a complicated structure and com- at Earth’s surface, distributed about equally between position.
Details
-
File Typepdf
-
Upload Time-
-
Content LanguagesEnglish
-
Upload UserAnonymous/Not logged-in
-
File Pages36 Page
-
File Size-