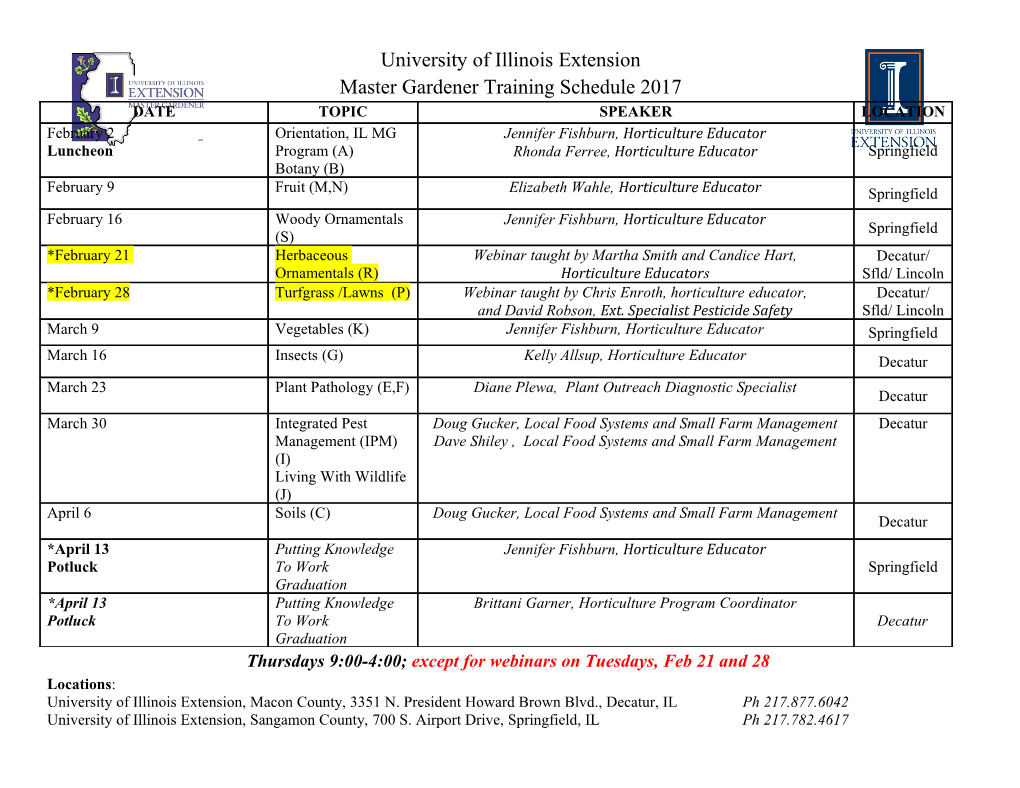
Holsapple et al.: Asteroid Impacts: Experiments and Scaling Laws 443 Asteroid Impacts: Laboratory Experiments and Scaling Laws K. Holsapple University of Washington I. Giblin Planetary Science Institute K. Housen The Boeing Company A. Nakamura Kobe University E. Ryan New Mexico Highlands University The present states of the small bodies of the solar system are largely an outcome of colli- sional processes. A rocky main-belt asteroid has endured a multitude of small and large cratering impacts; for example, estimates here show that one starting with a radius of 1 km has been shattered about five times every 106 yr and one with a radius of 10 km has been shattered about every 107 yr, or perhaps even more frequently in the past when collision rates were higher than they are now. All solar system bodies bear the scars and imprints of those impacts. Much has been learned about these topics since publication of the Asteroids II book (Fujiwara et al., 1989). Here we briefly review the previous wisdom, but primarily address new experiments, calcula- tions, and scaling methods. 1. INTRODUCTION nation of nuclear bombs down to those involved in the stat- ics of ordinary soil and rock mechanics. The results of a An understanding of collisional processes and collisional given impact are therefore very difficult to predict, although evolution is required in order to interpret observations of much progress has been made over the last few decades. the solar system. Although laboratory experiments and com- The outcome of a collision depends largely on the ratio puter simulations have provided many insights into these of the kinetic energy of the impactor to the mass of the im- processes, our understanding of energetic impacts is still pacted body, a specific energy, commonly denoted as Q. relatively primitive. Each new view of asteroids provided Two threshold values of Q are often defined, although the by spacecraft brings new surprises. For example, the sub- literature is not consistent in terminology or notation. Im- stantial regolith on a small body like Gaspra, the huge pacts with small values of Q form craters, but leave the tar- closely packed craters on Mathilde, and the block-strewn get body largely intact. Larger values of Q can shatter a surface of Eros were entirely unexpected. Nevertheless, body into numerous pieces. The specific energy to shatter, * experiments and modeling, guided by observations of as- QS, is defined as the threshold value for which the largest teroids, will allow us to deduce much about the collision remaining intact piece immediately following a collision has history of these bodies, including crater sizes and frequency, one-half the mass of the original body. We refer to it as the crater morphology, ejecta block distributions, regolith de- shattering energy. The shattered pieces may reaccumulate velopment, and the formation of asteroid families. or not, depending on their velocity relative to the escape * Impact processes are very complex and involve extreme velocity. A higher threshold QD is the specific energy such ranges of conditions. The initial coupling of energy from a that the largest object following reaccumulation is one-half high-speed impactor into another body can occur in micro- the mass of the original body. This is called the dispersion seconds, with the extreme pressures and temperatures suffi- energy. The term disruption energy is sometimes used in cient to melt and vaporize the target and projectile material. the literature for either of these thresholds, often in a ge- On large bodies, the latter stages of these processes can neric way for any significant breaking and/or dispersion. continue for hours and involve very low pressures. These Numerous unsolved problems remain for collisions in conditions range from those encountered in the initial deto- all three regimes. Important questions about cratering in- 443 444 Asteroids III clude the crater size; the shape; the amount of compaction; tioned above. The variety of physical material response is and the amount, velocity, and fate of ejected material. For enormous, and we have at best a very rough understanding shattering impacts, the distributions of velocity, size, shape, of relevant physical models. One must model the material and spin of the pieces, and the ultimate fate of those pieces, behavior during shock propagation; crushing of voids in the are still largely unknown. How many fragments remain in material; and failure, flow, and fracture. Important features place, how many are lofted and reaccumulate, and what is such as compaction, strain softening, nonlinearity, and hys- the structure of that modified body? What conditions de- teresis are seldom included. We have only a crude under- termine whether a body is shattered but basically intact, as standing of even the nature of these responses; even when Eros might be, or completely turned to rubble? Finally, for complicated mathematical models are hypothesized and * energy above QD, when the body is shattered and scattered, constructed, there is seldom enough data to calibrate them, significant questions remain about the size distribution of especially for three-dimensional states. Tests of sensitivi- the fragments, the largest piece, and their ultimate fate. ties to inputs are rarely made. As a result, code calculations There are three interrelated and complementary ap- must always be questioned, and even more so when few proaches to the study of these processes. All three approaches attempts are made to calibrate them against known experi- suffer from our lack of detailed knowledge about the ma- mental results. terials and structure of solar system bodies and asteroids. The third major approach uses scaling methods. Scaling In addition, each approach also has its own shortcomings. theories are developed to predict how collisional processes The first approach is to conduct laboratory experiments. will depend on the parameters of the problem, including the Laboratory methods use guns to launch a projectile into a size, impact velocity, gravity, and material type. They are target at speeds up to ~7 km/s, or explosive charges to simu- developed from considerations of similarity analysis applied late an impact. While experiments allow the study of actual to experimental results, from code calculations themselves, geological materials, their primary shortcoming is the in- and from observations of asteroids. However, scaling laws ability to use targets and projectiles of the size of interest. are based on assumptions about the importance of various Laboratory experiments are limited to samples of centimeter parameters. They always require some tie to experiments or size, while the asteroids range to many hundreds of kilo- calculations to determine unknown constants and can lead to meters in size. The response of a small target to impact is erroneous conclusions if important parameters are neglected dominated by its material strength, while that of a large as- or results are extrapolated into regimes of new physics. teroid is dominated by gravitational forces. For the predomi- Since the last contributions in Asteroids II, it has become nantly unidirectional gravity field that governs surface cra- clear that there are at least four major issues about the tering, the lack of sufficient gravity for the experimental mechanics of small-body impacts that have not been suffi- small targets can sometimes be overcome by performing ciently addressed. The first is the effect of substantial poros- experiments at high artificial gravity using a geotechnical ity. Twelve years ago there was conjecture about the exis- centrifuge, but that tool is of little use for the three-dimen- tence of rubble-pile asteroids [the term “rubble piles” was sional gravity fields dominating catastrophic disruptions. first introduced by Davis et al. (1977)], but no knowledge of Additional uncertainties are introduced by an inability to the effects of the implied porosity on the shock processes. perform experiments at velocities of several tens of kilome- There were neither analysis nor code calculations for the ters per second. Features that are important at low velocities, cratering and disruption of porous asteroids. Now the sci- such as projectile material and shape, probably are not sig- entific community seems to have reached consensus that nificant at high velocities. As a consequence of these limita- many and maybe even most large asteroids are reaccumu- tions, laboratory experiments can only probe a small part of lated rubble piles with possibly large porosity. The dis- the parameter space of interest. covery of low densities in C-type asteroids such as Mathilde The second approach is to use computer calculations and Eugenia strongly indicate high porosity. For example, based on the underlying physical principles. These meth- Mathilde has a bulk density of about 1.3 g/cm3, implying ods continue to evolve. Finite-difference, finite-element and, a porosity of 50%. Rocky asteroids are estimated to have over the last decade, smooth-particle-hydrodynamics (SPH) been shattered many times over the lifetime of the solar sys- methods have been particularly useful, giving efficient ways tem and may reaccumulate into a low-density state. Comets to study impact processes. As computer power grows in are also thought to be very low-density conglomerates of leaps and bounds, we can now calculate many more cases ice and dirt. in much less time. However, these methods are also lim- Porosity may be the dominant physical property
Details
-
File Typepdf
-
Upload Time-
-
Content LanguagesEnglish
-
Upload UserAnonymous/Not logged-in
-
File Pages20 Page
-
File Size-