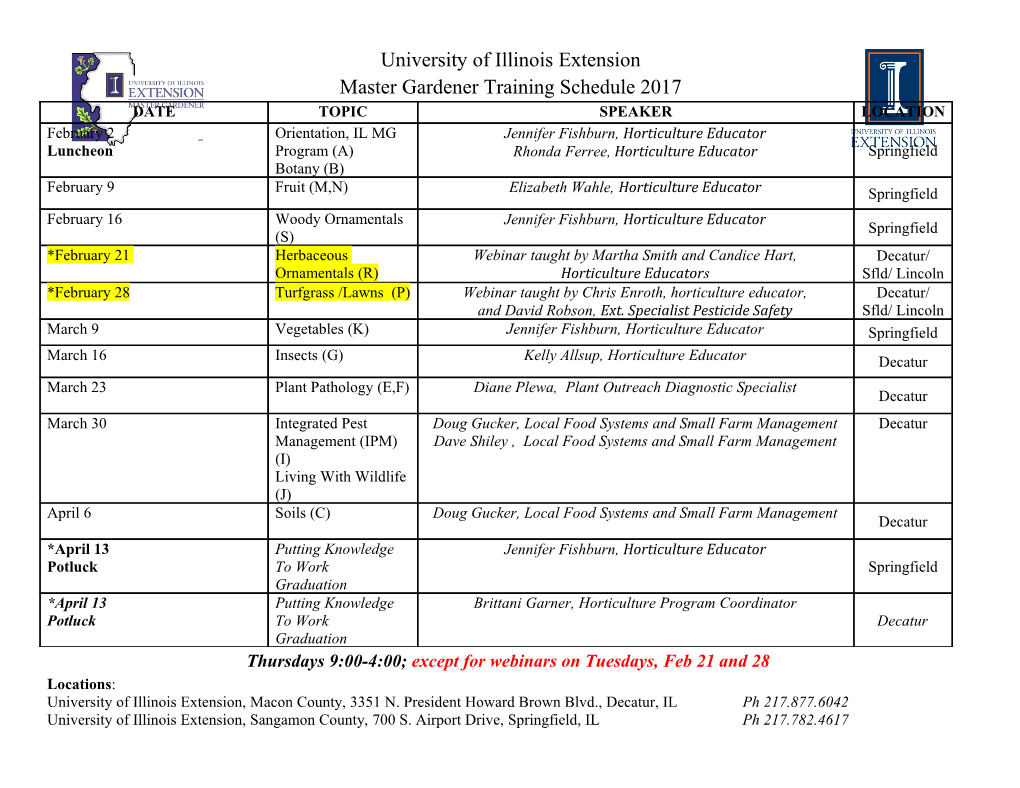
Downloaded from http://cshperspectives.cshlp.org/ on October 2, 2021 - Published by Cold Spring Harbor Laboratory Press Beyond DNA and RNA: The Expanding Toolbox of Synthetic Genetics Alexander I. Taylor, Gillian Houlihan, and Philipp Holliger Medical Research Council Laboratory of Molecular Biology, Cambridge CB2 0QH, United Kingdom Correspondence: [email protected] SUMMARY The remarkable physicochemical properties of the natural nucleic acids, DNA and RNA, define modern biology at the molecular level and are widely believed to have been central to life’s origins. However, their ability to form repositories of information as well as functional structures such as ligands (aptamers) and catalysts (ribozymes/DNAzymes) is not unique. A range of nonnatural alternatives, collectively termed xeno nucleic acids (XNAs), are also capable of supporting genetic information storage and propagation as well as evolution. This gives rise to a new field of “synthetic genetics,” which seeks to expand the nucleic acid chemical toolbox for applications in both biotechnology and molecular medicine. In this review, we outline XNA polymerase and reverse transcriptase engineering as a key enabling technology and summarize the application of “synthetic genetics” to the development of aptamers, enzymes, and nanostructures. Outline 1 Introduction 4 Nanoscale engineering using synthetic genetic polymers 2 Polymerase engineering for synthetic genetics 5 Concluding remarks: Worlds beyond RNA? 3 Evolving function using synthetic genetic References polymers Editors: Thomas R. Cech, Joan A. Steitz, and John F. Atkins Additional Perspectives on RNA Worlds available at www.cshperspectives.org Copyright # 2019 Cold Spring Harbor Laboratory Press; all rights reserved; doi: 10.1101/cshperspect.a032490 Cite this article as Cold Spring Harb Perspect Biol 2019;11:a032490 1 Downloaded from http://cshperspectives.cshlp.org/ on October 2, 2021 - Published by Cold Spring Harbor Laboratory Press A.I. Taylor et al. 1 INTRODUCTION this approach promises both new insights into the chemical boundary conditions of genetics as well as new tools to The capacity of DNA and RNA for accessible high-density study and modify biological processes. A prime example information storage and propagation separates nucleic ac- is the modification of nucleobases, utilizing alternative hy- ids from other sequence-defined biopolymers (including drogen bonding patterns, hydrophobic and/or geometric proteins and peptides). This provides both the medium compatibility, or metal ion chelation, toward an expansion and the mechanism for Darwinian evolution and is as of the genetic alphabet in vitro and in vivo, as well as yield- yet unmatched by any other polymer or other molecular ing secondary structure motifs beyond those of DNA and system. Indeed, DNA can store as much as 200 petabytes RNA (reviewed in Lee et al. 2018). Furthermore, in vitro of information per gram with high chemical stability. replication and evolution of nucleic acid variants compris- This function is underpinned by a unique chemistry in- ing backbone chemistries not found in nature (here referred cluding the polyanionic phosphodiester backbone, which to collectively as xeno nucleic acids [XNAs]) yields ligands dominates the physicochemical behavior and thereby (XNA aptamers) and enzymes (XNAzymes), as well as sim- decouples base sequence (i.e., information content) from ple nanostructures with novel properties including in- molecular properties and Watson–Crick base-pairing (a creased biostability (Pinheiro et al. 2012; Yu et al. 2012; combination of hydrogen-bonding and stacking interac- Taylor et al. 2015; 2016). Similarly, expansion of DNA mo- tions), which allows encoding and decoding of information lecular diversity (primarily through pyrimidine C5 substit- in a redundant fashion. uent chemistry) has accelerated aptamer discovery (Gold et al. 2010) and improved nucleic acid catalysts (Zhou et al. 1.1 Natural Chemical Variation 2016; Wang et al. 2018). Here, we provide an overview of the synthetic genetics toolbox, with a special focus on the Given the stringency of molecular requirements for func- impact of replacing the canonical ribofuranose sugar of tion, one might expect the chemical makeup of natural DNA and RNAwith synthetic congeners, informing broad- nucleic acids to be completely uniform. However, consid- er chemical possibilities for biotechnology and genetic erable chemical variation from the cognate DNA and RNA function (Fig. 1). chemistry is found in nature. Such variation is both diverse and widespread, including a range of epigenetic and other markers in both prokaryotic and eukaryotic DNA. Al- though most occur sporadically, some modifications can 2 POLYMERASE ENGINEERING FOR SYNTHETIC completely replace the canonical bases in some bacterio- GENETICS phage genomes (reviewed in Weigele and Raleigh 2016). High-fidelity polymerase enzymes catalyze synthesis and Arguably, such bacteriophages represent genetics based decoding of DNA sequence information with error rates as − on alternative, genetic polymers, suggesting that integration low as 1 × 10 7 coupled with an exquisite substrate specif- of expanded chemistry sets into living systems (xenobiol- icity enforced by both minor groove readout and active site ogy) may be possible (Herdewijn and Marlière 2009). geometrical constraints. This provides for both accurate fi In RNA, in addition to a diverse array of modi cations substrate selection and exclusion of damaged and/or non- found in transfer RNAs (tRNAs) (reviewed in Schimmel cognate nucleotides. Indeed, DNA polymerases must 2018), an expanding range of epigenetic messenger RNA stringently select cognate deoxyribonucleotide triphos- (mRNA) markers modulating translation initiation, RNA phates (dNTPs) from a pool that may contain between half-life, and even decoding by the ribosome have been 10- and 100-fold higher concentration of ribonucleotide discovered (reviewed in Meyer and Jaffrey 2017). triphosphates (NTPs) as incorporation of NTPs into the genome leads to genomic instability. The molecular basis of NTP discrimination lies in bulky amino acid side chains 1.2 Artificial Chemical Variation and Synthetic (e.g., tyrosine in the case of the archaeal polB family po- Genetics lymerases like Thermococcus gorgonarius [Tgo]: Y409) Beyond the natural variations, organic chemistry has ex- (Cozens et al. 2012), which excludes nucleotides with 2′- plored a much wider range of alternative backbones, sugar OH substituents from the polymerase active site. This congeners, and base chemistries with the aim to better de- “steric gate” residue has analogs in all DNA polymerase fine the key molecular parameters required for nucleic acid families and is paradigmatic of the mechanisms poly- function (Eschenmoser 1999). This has recently been ex- merases use to ensure substrate specificity, understanding tended to their potential for genetic information storage, of which will be key for progress in synthetic genetics propagation, and evolution. Termed “synthetic genetics,” (Fig. 1). 2 Cite this article as Cold Spring Harb Perspect Biol 2019;11:a032490 Downloaded from http://cshperspectives.cshlp.org/ on October 2, 2021 - Published by Cold Spring Harbor Laboratory Press Beyond DNA and RNA for Synthetic Genetics O O O O O O O O O O O Base Base Base Base Base Base HO F HO N3 HO NHNH2 HO F HO OOH O OH 2′ Fluoro 2′ Azido 2′ Amino FANA ANA TNA O O O O Base O O Base O O O O O Base Base Base Base OH OH HO O OH HO OH H3COCO OH HNA CeNA LNA/BNA 3′deoxy 3′OMe L-RNA HN Base O O O O Base O Base O O Base Base N O HO O O Base O OH OH HO HO OH – O NH O P BH3 AtNA PNA GNA xyNA HOMO-NA O Boranophosphate Figure 1. Chemical structures of unnatural nucleic acids investigated for synthetic genetics. Sugar modifications incorporated by polymerases are shown in red, whereas nucleic acids that currently cannot be synthesized by polymerases are shown in pink. Unnatural chemistries that do not hybridize to natural nucleic acids are shown in blue, and modifications to the phosphodiester backbone are shown in purple. 2.1 Engineering Primer-Dependent RNA 2.2 Engineering Modified DNA and RNA Polymerases Polymerases The comparable simplicity of such molecular checkpoints To incorporate 2′ modified nucleotide substrates, much has inspired efforts to engineer DNA polymerases into work has gone into engineering T7 RNA polymerase primer-dependent RNA polymerases (Houlihan et al. (T7RP) (e.g., for incorporation of dNTPs as well as ′ ′ 2017a). Replacement of the steric gate with less bulky amino 2 F- and 2 N3-pyrimidine triphosphates [YTPs]). One acids can improve NTP incorporation kinetics, up to levels such T7RP is available commercially as DuraScribe (Luci- comparable to dNTP incorporation kinetics by DNA poly- gen Corp.); it enables incorporation of 2′OMe-, 2′F-, and ′ ′ merases, but still precludes RNA synthesis beyond short 2 N3-YTPs or all four 2 OMe-NTPs provided GTP is oligomers. Indeed, a second checkpoint in the thumb also present to enable the initiation step (Burmeister et al. domain was recently discovered that enabled primer-de- 2005). pendent synthesis of longer RNAs (Cozens et al. 2012). DNA polymerases from the polA family have also Primer-dependent RNA synthesis has a number of appli- been extensively engineered for 2′-modified substituents. cations, as it obviates the need to initiate transcription with Phage display was adapted to evolve the Stoffel fragment a5′-pppG and allows a wide range of chemical groups to be of Taq polymerase for 2′OMe-CTP
Details
-
File Typepdf
-
Upload Time-
-
Content LanguagesEnglish
-
Upload UserAnonymous/Not logged-in
-
File Pages17 Page
-
File Size-