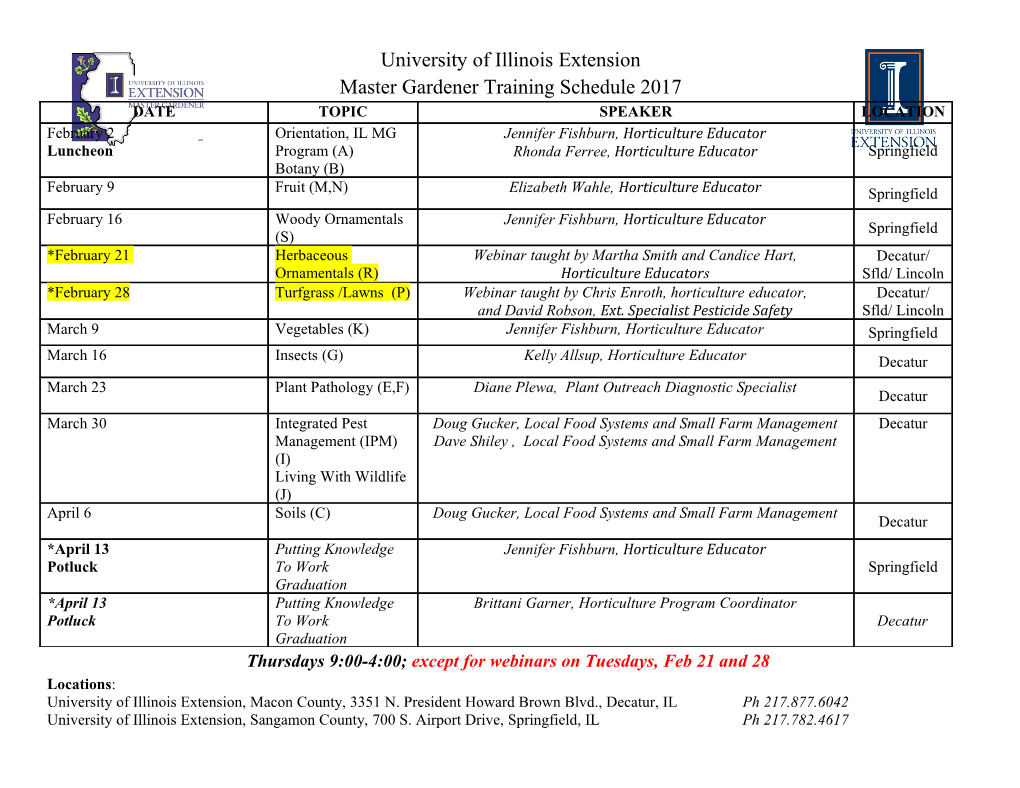
NEUTRON INDUCED FISSION FRAGMENT ANGULAR DISTRIBUTIONS AND MOMENTUM TRANSFER MEASURED WITH THE NIFFTE FISSION TIME PROJECTION CHAMBER by David Hensle c Copyright by David Hensle, 2019 All Rights Reserved A thesis submitted to the Faculty and the Board of Trustees of the Colorado School of Mines in partial fulfillment of the requirements for the degree of Doctor of Philosophy (Applied Physics). Golden, Colorado Date Signed: David Hensle Signed: Dr. Uwe Greife Thesis Advisor Golden, Colorado Date Signed: Dr. Uwe Greife Professor and Head Department of Physics ii ABSTRACT Nuclear fission is the process by which a large nucleus splits into two heavy fragments and is often induced by an incident neutron. Understanding the probability by which an incident neutron will cause fission, i.e. the neutron induced fission cross section, is an important input into fission applications such as nuclear energy and stockpile stewardship. The Neutron Induced Fission Fragment Tracking Experiment (NIFFTE) Collaboration built a fission time projection chamber (fissionTPC) to study the fission process in a novel way in the hopes of achieving unprecedented precision on fission cross section measurements. With the fissionTPC’s ability to do three-dimensional tracking of fission fragments and other ionizing radiation, systematics of previous cross section measurements using fission chambers can be further explored. Because the fissionTPC records a wealth of data for every fission event, other physics can be measured concurrently with the cross section. In particular, fission fragment angular distributions and the linear momentum transferred from the incident neutron to the target nucleus as a function of incident neutron energy from 130 keV to 250 MeV will be the focus of this work. Angular anisotropy values for 235U and 238U and neutron linear momentum transfer for 235U, 238U, and 239Pu will be presented. iii TABLE OF CONTENTS ABSTRACT ......................................... iii LISTOFFIGURES .....................................vii LISTOFTABLES ......................................xiv ACKNOWLEDGMENTS ..................................xv CHAPTER 1 NUCLEAR FISSION BACKGROUND . 1 1.1 FissionBasics .................................... 1 1.2 NeutronInducedFission ............................. .7 1.2.1 NIFFTECrossSectionFormulation . 10 1.3 FissionFragmentAngularAnisotropy . .... 12 1.4 NeutronLinearMomentumTransfer . 14 1.5 Motivation for Precise Fission Measurements . .........16 CHAPTER 2 NIFFTE TIME PROJECTION CHAMBER . 18 2.1 Fission Time Projection Chamber Hardware and Operation . .........18 2.1.1 ElectronicsandTriggering . 20 2.1.2 FissionTPCFillGas ............................ 24 2.2 ActinideTargets .................................. 28 2.3 NeutronSourceandTimeofFlightMeasurement . ..... 32 2.3.1 CarbonFilterData............................. 34 2.3.2 NeutronBeamWraparound . 35 CHAPTER 3 TPC DATA RECONSTRUCTION AND CORRECTIONS . 37 iv 3.1 DataReconstruction: SignalstoTracks . ........37 3.2 NeutronTimeofFlightReconstruction . ..... 42 3.3 CorrectionstoData............................... 43 3.3.1 Californium-252Measurement . 44 3.3.2 PadSaturation ............................... 45 3.3.3 ElectronDriftSpeed . 50 3.3.4 ElectronDiffusionAnisotropy . 52 3.4 CorrectionParametersAppliedtoTargets . ....... 59 CHAPTER 4 NEUTRON LINEAR MOMENTUM TRANSFER . 61 4.1 Measuring Fragment Folding Angle Distributions . .........62 4.2 SimulationofFragmentOpeningAngles . ..... 64 4.3 LinearMomentumTransferError . .... 69 4.4 Linear Momentum Transfer Results and Discussion . .........75 CHAPTER 5 FISSION FRAGMENT ANGULAR ANISOTROPY . 80 5.1 MeasuredAnisotropy............................... 80 5.2 UncertaintyBudget................................ 84 5.3 MultipleAnisotropyMeasurements . ..... 92 5.4 MeasuredAnisotropyResults . 96 CHAPTER 6 SUMMARY AND OUTLOOK . 102 REFERENCESCITED .................................. 106 APPENDIX A CONVERSION BETWEEN LAB FRAME AND CENTER OF MASSFRAME .............................. 117 APPENDIX B DERIVATION OF THE ELECTRON DIFFUSION ANISOTROPY CORRECTION .............................. 120 v APPENDIXCTABULATEDDATA . 124 vi LIST OF FIGURES Figure 1.1 A cartoon depiction of neutron induced fission of 235U. ...........2 Figure 1.2 A visual representation of each of the terms in 1.1 for the semi-empirical mass formula describing the binding energy associated withtheliquiddropmodel.. .3 Figure 1.3 (a) Potential energy curves as a function of L2 deformation (minimized with respect to the L4 component) for different nuclei calculated by the liquid drop model (dashed curves) and the liquid drop model with shell corrections added (solid curves). Figure from . (b) Nuclear deformation described by the second and fourth order Legendre polynomial components,from. ...............................5 Figure 1.4 A geometrical interpretation of the partial cross sections for thermal neutrons incident on a 235Utarget. ......................8 Figure 1.5 Fission fragment mass yields vary as a function of incident neutron energy where symmetric fission is more preferred at high incident neutronenergies.Figurefrom.. 9 Figure 1.6 Depiction of the total angular momentum J and projection M and K onto the beam axis and symmetry axis, respectively. From these quantum numbers, the angular distribution of the fission fragments can bepredicted. ................................. 12 Figure 2.1 Schematic representation of the fission time projectionchamber. 19 Figure 2.2 (a) A schematic representation of an alpha particle’s ionization charge cloud being drifted to the anode and amplified by the MICROMEGAS. (b) A projection of a charge cloud onto the hexagonal readout pads on theanodes.................................... 20 Figure 2.3 (a) A picture of one of the two highly segmented anodes. (b) The fissionTPC fully assembled with all of the readout electronics attached. The drift chamber is 15 cm in diameter and the central cathode is 5.4 cm fromeachanode. ............................. 21 vii Figure 2.4 (Left) Picture of an EtherDAQ card. (Center) A schematic layout of an EtherDAQ card containing the analog amplifiers on the bottom where the input signal enters, and the digital electronics and fiber readout on top. (Right) Work flow diagram of the FPGA firmware that controls thedataflowfortriggeringandprocessing. 22 Figure 2.5 A representation of how low energy Argon recoils can produce more ionization density in the electron avalanche than a fission fragment and causesparking.Source.. 27 Figure 2.6 (a) A picture of the Plutonium side of the 239Pu/235U thick backed aluminum target before insertion into the fissionTPC. (b)Start vertices for alpha particles emitted from a thick target with 235U on each side. Contamination of small quantities of 239Pu can be seen on the cathode. 29 Figure 2.7 List of targets from which neutron induced fission data was taken. Actinides include 235U (blue), 238U (purple), and 239Pu(green). Aluminum target holders are 2cm in diameter and actinide deposits are drawnroughlytoscale. 31 Figure 2.8 On overview of the Los Alamos Neutron Science Center. The location of the 90L WNR beamline where the TPC is operated is circled in red. 32 Figure 2.9 Neutron time of flight spectrum for fission from a U235 target. The insert shows the photofission peak and fit to determine a time of flight resolution. Neutron wraparound is shown as the red line. ..... 33 Figure 2.10 (a) Inelastic neutron scattering cross section for Carbon-12 showing strong resonance feature at 2.078 MeV, from ENDF/B-VIII . (b) Neutron time of flight spectrum as measured by the fissionTPC showing location of the Carbon-12 resonance at around 400 ns. .....34 Figure 2.11 (a) The end of the last micropulse is fit with a sum of two decaying exponentials. This fit is then “folded” to get a prediction for the number of wraparound neutrons. (b) Percent of fission events caused by wraparound neutrons for a 235U target as a function of neutron energy calculated by taking the ratio of counts above and below the red wraparoundlineinFigure2.9. 36 Figure 3.1 Signals from an alpha particle and the subsequent differentiation used in the digit forming process. Considering all of the pads have signals occurring at almost the exact same time, this set of signals corresponds to an alpha particle that was emitted very parallel to the anode plane, equating to a polar angle of cos(θ)veryclosetozero. 38 viii Figure 3.2 A typical fission event from a thin carbon backed target. Along with the back to back fission fragments, small tracks from inelastic scattering of neutrons off protons in the gas are also visible. The red lines through the digits are the assigned tracks. Color scale denotes the ADC (charge)valueofeachdigit. 39 Figure 3.3 Track length vs total track energy is a commonly used parameter space toperformparticleidentification. 41 Figure 3.4 Raw high speed cathode signal (blue) induced from a fission event and its trapezoidal filtered counterpart (red). Where a straight line fit to the rising edge of the filtered signal intersects with 0 is taken as the timeofflightfortheevent. 43 Figure 3.5 (a) Fission fragment start vertices and (b) Length vs ADC from the 252Cf source taken at the 210V MICROGMEGAS setting . 44 Figure 3.6 (a) Saturated signal readouts from the pads for a fission fragment directed towards the anode. (b) A cut on the end portion of a charge cloud projected on the pads showing the hole created by the saturated signals. ....................................46 Figure 3.7 ADC vs cos θ for 252Cf fragment events at the four different gain
Details
-
File Typepdf
-
Upload Time-
-
Content LanguagesEnglish
-
Upload UserAnonymous/Not logged-in
-
File Pages146 Page
-
File Size-