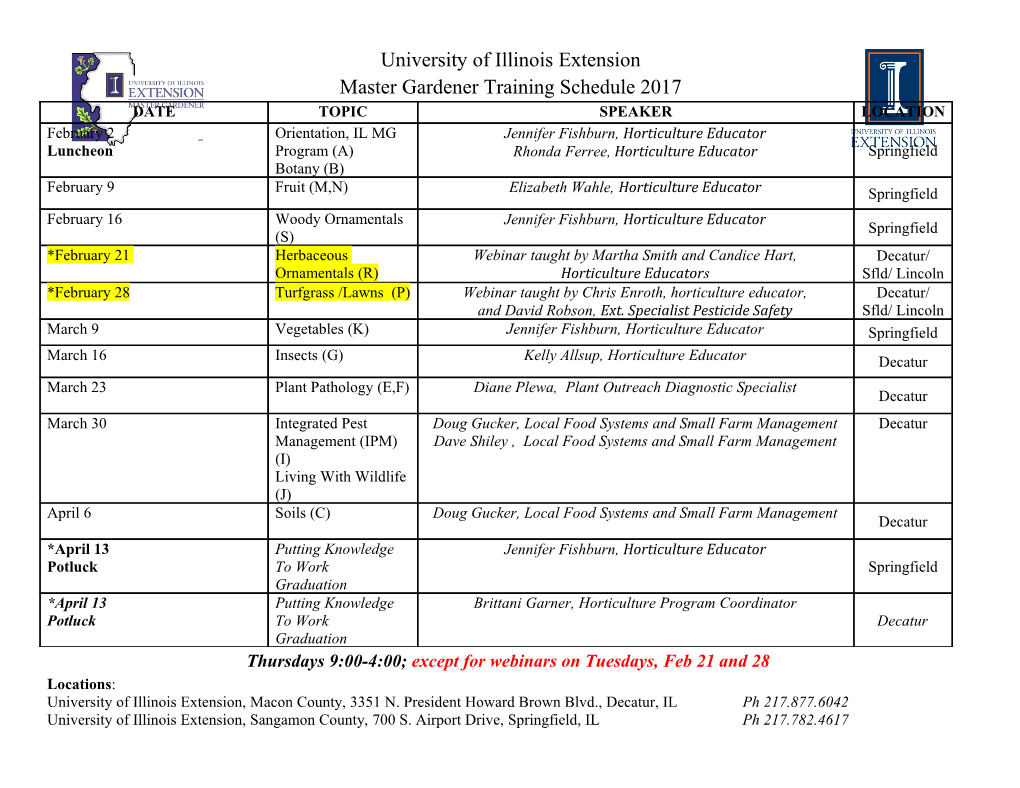
Shining the Light on the Structural Color of Tenacibaculum Ectocooler Chi Nguyen Microbial Diversity, Marine Biology Laboratory 2017 Introduction The use of color is ubiquitous through out all three domains of life and shown to play many important roles. Colors in nature are categorized into two groups, pigmented and structural color (Figure 1). Pigmented colors result from the selective absorption and reflection of colors at specific wavelengths. The most well characterized pigmented colors include chlorophyll and !-carotene that selectively reflect wavelengths in the green and orange spectrum, respectively (Figure 1). In contrast to pigmented color, structural colors do not result from selective absorption of colors but rather from non-specific and diffused diffraction of light at multiple wavelengths. This phenomenon occurs when light crosses between mediums of differing refractive indices (Figure 2), typically from air onto a surface comprised of regularly placed materials1. Changing in color by altering the viewing perspective, also called iridescence, is a defining characteristic of structural color and results from differential interactions of wavelengths (Figure 2). Colors in Nature Pigmented Color Structural Color Chlorophyll Figure 1. Colors in nature. The physics of pigmented versus structural color and examples of where they occur in nature. The usage of pigmented colors in animals for mate attraction and for evading predation has been well documented. It is thought that animals use structural colors for similar function. In contrast to animals, the function of bacteria iridescence is not well understood. Studies on the Himalayan tropical plant, Begonia pavonina, suggest that its blue-ish iridescence is a result of more selective reflection of blue light and may play a role in photo-protection against damaging UV light2. The photoprotective function of iridescence, if true, may also play important roles in bacterial iridescence such as Tenacibaculum Ectocoolest, discovered on surface marine water at Woods Hole by Rebecca Mickols. Since then, several other species of Tenacibaculum have been discovered my members of the 2017 Microbial Diversity students on sea surface of grass as well as in symbiotic relationship sea corals. Similarly,The Tenacibaclum Physics has also beenof isolatedIridescence from the surfaces of marine sea sponges near Japan3. Figure 2. Wave function and iridescence. (Left) Light waves can interact constructively, destructively, or a combination of both. Iridescence occurs because the sum of lights wavelengths changes depending on viewing angle. (Right) An schematic of the iridescent layer of bird feathers. While little known about the function of structural colors in bacteria, it has been shown that cell-cell organization plays key roles in iridescence. Studies in the bacterium Cellulophaga lytica illustrates that ordered cell-cell contact is key in iridescence and disruption of ordered cell-cell “lattice” results in loss of iridescence4. These results are consistent with studies in animals and plants using electron microscopy (EM) and small- angle X-ray scattering (SAXs) that illustrate highly ordered cell-cell arrays in iridescent birds, insects, and plants5,6 (Figure 3). At the molecular level, iridescence in these animals and plants are the result of highly ordered polysaccharides, such as chitin and cellulose, or proteins, including keratin and collagen5,6. Both of these iridescent biomaterials are often found in complex with structural proteins that may aid in their organization5,6. In contrast to the characterized iridescent biomaterials in animals and plants, little is known about what macromoleculesOrganization comprise iridescence of in bacteria.Iridescence As such, the goal of my mini project is to identify the macromolecule(s) that make up iridescence in bacteria using T. ectocooler as a model organism. Figure 3. Examples of highly ordered cells in iridescent plant, animals, and the bacterium Cellulophaga lytica (last two panels). Vinod Saranathan et al. Journal of Royal Society 2012. ResultsZhonge Gu andet at. Chemical Discussion Review Society 2012. Rosenfeld E. et al. Applied and Environmental Microbiology 2012. Whole Cell Assay Structural colors in plants and animals are comprised of the polysaccharides chitin and cellulose and the proteins keratin and collagen. Neither collagen or keratin are produced in bacteria, therefore it is more likely that bacterial iridescence may be comprised of polysaccharides (Figure 4). Production of complex sugar in bacteria are biosynthesized by transmembrane enzymes7,8, therefore it is likely that the sugar is tethered to the bacterial membrane. Given this rationale, I designed two whole cell assays to test the polysaccharide composition of T. ectocooler. Whole Cell Assay: Rationale Figure 4. Predicted iridescent macromolecules and their localization in T. ectocooler. Keratin and collagen are not made in bacteria, therefore it is likely that T. ectocooler iridescence is made of Iridescent protein polysaccharides. Polysaccharide biosynthesis Structural protein occurs on bacterial membrane, therefore the Iridescent sugar sugar is likely attached. The iridescent layer is likely uniformed, therefore it is likely intracellular, most likely the periplasm. 1.! Keratin and collagen are not made in bacteria ! likely a sugar 2.! Polysaccharide biosynthetic enzyme are located at the membrane ! likely attached 3.! Uniform lattice formation ! likely intracellular To test whether polysaccharides composition affect iridescence, I streaked T. ectocooler on plates containing lysozyme, cellulase, chitinase, and sucrase in combination with proteinase K and toluene (Figure 5). As compared to untreated cells and protienase K alone, cells grown on plates containing lysozyme, chitinase, and sucrase displayed a decrease in iridescence. The addition of toluene, which as been shown to permeablize cells, by itself or in combination with digestive enzymes lead to further disruption of iridescence in T. ectocooler (Figure 5). These Wholeresults links Cell structural Assay integrity and polysaccharide composition with T. ectocooler iridescence. 30°C + Proteinase K Observe iridescence +Polysaccharide Digestingdigesting enzymesEnzymes and Iridescence Polysaccharide Sugar monomer/oligomer Toluene Untreated Proteinase K Lysosyme Cellulase Chitinase Sucrase - + Figure 5. Colony iridescence assay in the presence of sugar digesting enzymes. (Top) Experimental setup for colony iridescence assay. (Bottom) The results of the colony iridescence assay. The addition of toluene, lysozyme,Toluene, chitinase, and lysozyme, sucrose led to decreaseschitinase, in colony and iridescence sucrase. ! decreases in iridescence To further investigate the polysacharride composition of T. ectocooler, I developed a second assay in which I grew this bacteria in liquid culture to stationary phase, incubated them with digestiveIs there enzymes, a change treated in the the cellspolysaccharide with toluene, and composition? stained for polysaccharide composition using the WGA and ConA lectin (Figure 6). The WGA-Alexa488 and ConA- Texas Red conjugates were used to stain for N-acetyl-glucosamine and mannose/glucose, respectively. It is likely, that digestion with these polysaccharide-digesting enzymes will lead to higher staining intensity. Cells left untreated or with proteinase K (data not shown) alone showed little to no staining with either WGA or ConA (Figure 6). Whole Cell Assay + proteinase K + Polysaccharide digesting enzymes Staining Requires Disruption of Outer Polysaccharide Sugar monomer/oligomer Membrane Lectin Overlay Toluene Toluene WGA lectin: N-acetylglucosamine - ConA lectin: Mannose/glucose N Acetylglucosamine + N Acetylglucosamine + Mannose/Glucsoe WGA stains for peptidoglycan Figure 6. Whole cell assay in liquid culture. (Top)ConA Experimental has low background setup. (Bottom) Images of cells treated and untreated by toluene and stained with WGA and ConA imaged under bright field (Left column), with filters set at 490 nm absorbance and 525 nm emission for WGA and 596 nm absorbance and 620 nm emission for WGA (Middle column), and the overlay of the two images (Right column). The top row are untreated cells. The bottom two rows are cells treated with toluene and proteinease K. The addition of toluene alone or in combination with proteinase K resulted in WGA staining but no ConA staining. The presence of WGA staining in permeablized cells and absence of polysaccharide-digesting enzymes suggest this lectin stains for the naturally occurring sugar components of T. ectocooler, most likely the N-acetyl-glucosamine of the cell wall. WGA may also bind to other polysaccharides that may play a role in iridescence but the high background prohibits further use of this lectin for additional investigation of T. ectocooler sugar composition in response treatment with sugar-digesting enzymes. In contrast, ConA displays low background and was used in to test the effect of the addition of cellulase, chitinase, and sucrose. In the presence of toluene, proteinase K, and sugar- digesting enzymes, T. ectocooler displayed significant staining for ConA (Figure 7). This suggests that digestion with these enzymes lead to changes in polysacharride composition, specifically depolymerization of complex sugars. In combination, the results of these two assays illustrates that the T. ectocooler structural integrity and polysaccharides influences iridescence. These studies provides the first insights into the molecular
Details
-
File Typepdf
-
Upload Time-
-
Content LanguagesEnglish
-
Upload UserAnonymous/Not logged-in
-
File Pages15 Page
-
File Size-