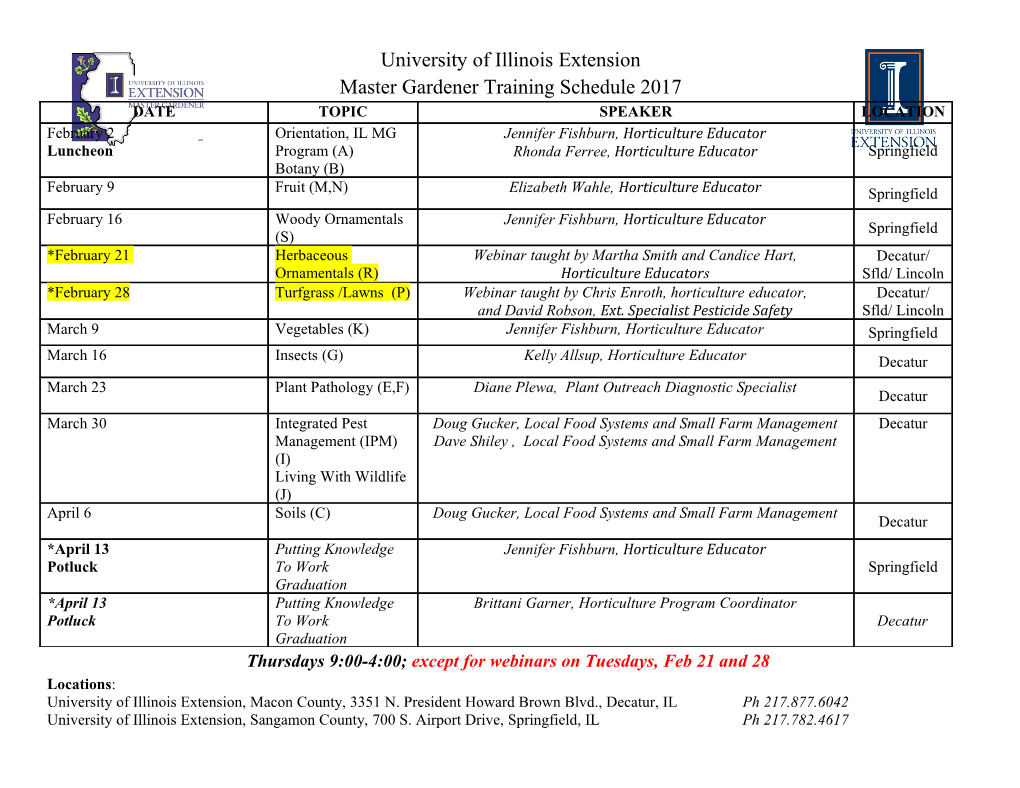
1 A Stochastic Model for Electron Transfer in Bacterial Cables Nicolo` Michelusi, Sahand Pirbadian, Mohamed Y. El-Naggar and Urbashi Mitra Abstract—Biological systems are known to communicate by network is described, and aspects such as reliability and speed diffusing chemical signals in the surrounding medium. However, of convergence of consensus are investigated. In [14], [15], a most of the recent literature has neglected the electron transfer new molecular modulation scheme for nanonetworks is pro- mechanism occurring amongst living cells, and its role in cell- cell communication. Each cell relies on a continuous flow of posed and analyzed, based on the idea of time-sharing between electrons from its electron donor to its electron acceptor through different types of molecules in order to effectively suppress the electron transport chain to produce energy in the form of the interference. In [16], an in-vitro molecular communication the molecule adenosine triphosphate, and to sustain the cell’s system is designed and, in [17], an energy model is proposed, vital operations and functions. While the importance of biological based on molecular diffusion. electron transfer is well-known for individual cells, the past decade has also brought about remarkable discoveries of multi- While communication via chemical signals has been the fo- cellular microbial communities that transfer electrons between cus of most prior investigations, experimental evidence on the cells and across centimeter length scales, e.g., biofilms and multi- microbial emission and response to three physical signals, i.e., cellular bacterial cables. These experimental observations open sound waves, electromagnetic radiation and electric currents, up new frontiers in the design of electron-based communications suggests that physical modes of microbial communication networks in microbial communities, which may coexist with the more well-known communication strategies based on molecular could be widespread in nature [18]. In particular, commu- diffusion, while benefiting from a much shorter communication nication exploiting electron transfer in a bacterial network delay. This paper develops a stochastic model that links the has previously been observed in nature [19] and in bacterial electron transfer mechanism to the energetic state of the cell. colonies in lab [20]. This multi-cellular communication is The model is also extensible to larger communities, by allowing usually triggered by extreme environmental conditions, e.g., for electron exchange between neighboring cells. Moreover, the parameters of the stochastic model are fit to experimental data lack of electron donor (ED) or electron acceptor (EA), in turn available in the literature, and are shown to provide a good fit. resulting in various gene expression levels and functions in different cells within the community, and enables the entire community to survive under harsh conditions. Electron transfer I. INTRODUCTION is fundamental to cellular respiration: each cell relies on a Biological systems are known to communicate by diffusing continuous flow of electrons from an ED to an EA through chemical signals in the surrounding medium. One example is the cell’s electron transport chain (ETC) to produce energy in quorum sensing [2]–[4], where the concentration of certain sig- the form of the molecule adenosine triphosphate (ATP), and to nature chemical compounds emitted by the bacteria is used to sustain its vital operations and functions. This strategy, known estimate the bacterial population size, so as to simultaneously as oxidative phosphorylation, is employed by all respiratory activate a certain collective behavior. More recently, molecular microorganisms. In this regard, we can view the flow of one communication has been proposed as a viable communication electron from the ED to the EA as an energy unit which is har- scheme for nanodevices and nanonetworks, and is under vested from the surrounding medium to power the operations IEEE standards consideration [5]. The performance evaluation, of the cell, and stored in an internal ”rechargeable battery” optimization and design of molecular communications systems (energy queue, e.g., see the literature on energy harvesting for arXiv:1410.1838v2 [cs.ET] 20 Feb 2015 opens up new challenges in the information theory [6]–[9]. The wireless communications and references therein [21]–[23]). achievable capacity of the chemical channel using molecular While the importance of biological electron transfer and communication is investigated in [10], [11], under Brownian oxidative phosphorylation is well-known for individual cells, motion, and in [12], under a diffusion channel. In [13], a new the past decade has also brought about remarkable discov- architecture for networks of bacteria to form a data collecting eries of multi-cellular microbial communities that transfer electrons between cells and across much larger length scales N. Michelusi and U. Mitra are with the Ming Hsieh Department of Electrical Engineering, University of Southern California, Los Angeles, USA; than previously thought [24]. Within the span of only a few M. Y. El-Naggar and S. Pirbadian are with the Department of Physics and years, observations of microbial electron transfer have jumped Astronomy, University of Southern California, Los Angeles, USA; emails: from nanometer to centimeter length scales, and the structural fmichelus,spirbadi,mnaggar,[email protected] N. Michelusi and U. Mitra acknowledge support from one or all of basis of this remarkably long-range transfer has evolved from these grants: ONR N00014-09-1-0700, CCF-0917343, CCF-1117896, CNS- recently discovered molecular assemblies known as bacterial 1213128, AFOSR FA9550-12-1-0215, and DOT CA-26-7084-00. S. Pirbadian nanowires [24]–[26], to entire macroscopic architectures, in- and M. Y. El-Naggar acknowledge support from NASA Cooperative Agree- ment NNA13AA92A and grant DE-FG02-13ER16415 from the Division of cluding biofilms and multi-cellular bacterial cables, consisting Chemical Sciences, Geosciences, and Biosciences, Office of Basic Energy of thousands of cells lined up end-to-end in marine sediments Sciences of the US Department of Energy. N. Michelusi is in part supported [19], [27] (see Fig. 1). Therein, the cells in the deeper regions by AEIT (Italian association of electrical engineering) through the research scholarship ”Isabella Sassi Bonadonna 2013”. of the sediment where the ED is located extract more electrons, Parts of this work have appeared in [1]. while the cells in the upper layers, where Oxygen (an EA) is 2 with respect to molecular diffusion is that the electron is both an energy carrier involved in the energy production for the cell to sustain its functionalities, and an information carrier, which enables the transport of information between nanodevices, thus introducing additional constraints in the encoded signal. Electron-based communication presents significant advan- tages, as discussed above, but this phenomenon also raises new intriguing questions. While a single cell can extract enough free energy to power life’s reactions by exploiting the redox potential difference between ED oxidation and EA reduction, how can the same potential difference be used to power an Figure 1. Fluorescent image of filamentous Desulfobulbaceae. Bacterial cells entire multicellular assembly such as the Desulfobulbaceae are aligned to form the cable, which couples Oxygen reduction at the marine sediment surface to sulphide reduction in deeper anoxic layers by transferring bacterial cables [19]? Specifically, can intermediate cells sur- electrons along its length. From [19]. vive without access to chemical ED or EA, by exploiting the potential difference between cells in the deeper sediment more abundant, have a heightened transfer of electrons to the (sulfide oxidizers) and cells in the oxic zone (oxygen reduc- EA. The survival of the whole system relies on this division ers)? For a cable consisting of thousands of cells this appears of labor, with the intermediate cells operating as ”relays” of unlikely, since the free energy available for an intermediate electrons to coordinate this collective response to the spatial cell is inversely proportional to the total number of cells. Are separation of ED and EA. It is worth noticing that other additional, yet unknown, electron sources and sinks necessary biological cable-like mechanisms exist in nature, enabling cell- to maintain the whole community? These questions necessitate cell communication: tunneling nanotubes connect two animal flexible models that analyze emerging experimental data in or- cells for transport of organelles and membrane vesicles and der to elucidate the energetics of individual cells, as presented create complex networks of interconnected cells [28]; in the here, and, eventually, whole bacterial cables or biofilms. bacterial world, Myxococcus xanthus cells form membrane tubes that connect cells to one another in order to transfer In order to enable the modeling and control of such micro- outer membrane content [29]. bial communications network and guide future experiments, in These experimental observations raise the possibility of an this paper we set out to develop a stochastic queuing theoretic electron-based communications network in microbial commu- model that links electron transfer to the energetic state of nities, which may coexist with the more well-known communi- the cell (e.g., ATP concentration or energy charge potential). cation strategies based on molecular
Details
-
File Typepdf
-
Upload Time-
-
Content LanguagesEnglish
-
Upload UserAnonymous/Not logged-in
-
File Pages15 Page
-
File Size-