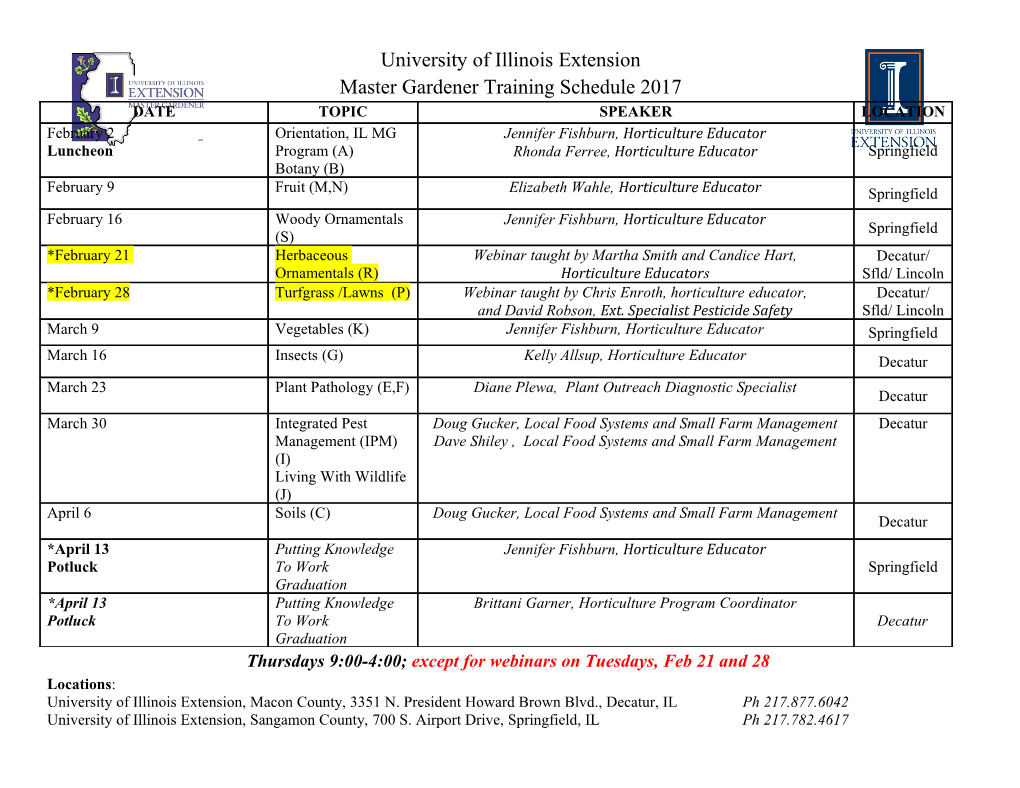
Coherent microwave photon mediated coupling between a semiconductor and a superconductor qubit P. Scarlino1†∗, D. J. van Woerkom1y, U. C. Mendes2, J. V. Koski1, A. J. Landig1, C. K. Andersen1, S. Gasparinetti1, C. Reichl1, W. Wegscheider1, K. Ensslin1, T. Ihn1, A. Blais2;3 and A. Wallraff1 1Department of Physics, ETH Zurich,¨ CH-8093 Zurich,¨ Switzerland 2Institut quantique and Department´ de Physique, Universite´ de Sherbrooke, Sherbrooke, Quebec´ J1K 2R1, Canada 3Canadian Institute for Advanced Research, Toronto, ON, Canada y These authors contributed equally to this work. gate voltages [22] and by fermionic cavities [23]. An alterna- ∗ To whom correspondence should be addressed. tive approach which allows for long-range qubit-qubit inter- action, inspired by superconducting circuit quantum electro- Semiconductor qubits rely on the control of charge and dynamics (QED) and recently explored also for semiconduc- spin degrees of freedom of electrons or holes confined in tor QDs [24–26], is to use microwave photons confined in quantum dots (QDs). They constitute a promising ap- superconducting resonators to mediate coupling between dis- proach to quantum information processing [1, 2], comple- tant qubits. In this approach, the microwave resonator not mentary to superconducting qubits [3]. Typically, semi- only acts as a quantum bus, but also allows for quantum non- conductor qubit-qubit coupling is short range [1, 2, 4, 5], demolition qubit readout [12–14]. effectively limiting qubit distance to the spatial extent With the well established strong coupling of supercon- of the wavefunction of the confined particle, which rep- ducting qubits to microwave resonators [6] and the recently resents a significant constraint towards scaling to reach achieved strong coupling to charge states in semiconductor dense 1D or 2D arrays of QD qubits. Following the suc- double dot structures [7, 8], it is now possible to create a mi- cess of circuit quantum electrodynamics [6], the strong crowave photon-based interface between superconducting and coupling regime between the charge [7, 8] and spin [9–11] semiconducting qubits mediated by a joint coupling resonator. degrees of freedom of electrons confined in semiconduct- A similar strategy has been explored in hybrid structures in- ing QDs interacting with individual photons stored in a terfacing a transmon qubit with excitations of a spin-ensemble microwave resonator has recently been achieved. In this of NV centers in diamonds [27–29] and of collective spins letter, we demonstrate coherent coupling between a super- (magnons) in ferromagnets [30–32]. Furthermore, direct cou- conducting transmon qubit and a semiconductor double pling between a superconducting flux qubit and an electron quantum dot (DQD) charge qubit mediated by virtual mi- spin ensemble in diamond was investigated [33]. In these crowave photon excitations in a tunable high-impedance works the strong coupling regime was achieved with ensem- SQUID array resonator acting as a quantum bus [12–14]. bles, for which the coupling strength scales with the square The transmon-charge qubit coherent coupling rate ( 21 root of the number of two-level systems interacting with the MHz) exceeds the linewidth of both the transmon ( ∼0:8 resonator mode. MHz) and the DQD charge ( 3 MHz) qubit. By tuning∼ Here, we explore the coupling of the charge degree of free- the qubits into resonance for∼ a controlled amount of time, dom of a single electron confined in a double quantum dot we observe coherent oscillations between the constituents (DQD) to a superconducting transmon qubit in the circuit of this hybrid quantum system. These results enable a new QED architecture [6]. To perform our experiments, we in- class of experiments exploring the use of the two-qubit in- tegrate four different quantum systems into a single device: teractions mediated by microwave photons to create en- a semiconductor DQD charge qubit, a superconducting qubit, tangled states between semiconductor and superconduct- and two superconducting resonators [see Fig. 1(a)]. One res- ing qubits. The methods and techniques presented here onator acts as a quantum bus between the superconducting are transferable to QD devices based on other material and the semiconductor qubits and the other one as a readout arXiv:1806.10039v1 [quant-ph] 26 Jun 2018 systems and can be beneficial for spin-based hybrid sys- resonator for the superconducting qubit. In this way, the func- tems. tionality for qubit readout and coupling is implemented using Single electron spins confined in semiconductor quantum two independent resonators at different frequencies, allowing dots (QDs) can preserve their coherence for hundreds of mi- for more flexibility in the choice of coupling parameters and croseconds in 28Si [15, 16] and have typical relaxation times reducing unwanted dephasing due to residual resonator pho- of seconds [17, 18]. This property can be explored, for ex- ton population [34]. A simplified circuit diagram of the de- ample, to build memories for quantum information proces- vice is shown in Fig. 1(f). The superconducting qubit is of sors in hybrid architectures combining superconducting qubits transmon type and consists of a single superconducting alu- and spin qubits. Strategies to interconnect semiconductor minum (Al) island shunted to ground via a SQUID (orange in qubits include the control of short-range interactions through Fig. 1). The transmon charging and Josephson energies are 0 the direct overlap of electronic wavefunctions [1, 4], the di- Ec=h 243 MHz and E =h 30 GHz, respectively (see ∼ J ∼ rect capacitive coupling between QDs [5], enhanced by float- Methods for more information). The transition frequency !tr ing metallic gates [19], shuttling of electrons between dis- between its ground state g and excited state e is adjusted tant QDs by surface acoustic waves [20, 21], by time-varying by using the magnetic fluxj generatedi in the transmonj i SQUID 2 (a) (b) 500 µm 100 µ m (c) (d) (e) (f) 1 µm 500 5µm nm coil 50 µm Transmon Squid Array 50 Ω Drive line resonator DQD S D FIG. 1. Sample and simplified circuit diagram. (a) False color optical micrograph of the device showing the substrate (dark gray), the Al superconducting structures forming the groud plane (light gray), the DQD Au gate leads (yellow), the SQUID array resonator (red), its microwave feedline (green), the single island transmon (orange), its readout 50 Ω coplanar waveguide resonator (blue) and the flux line (purple). (b) Enlarged view of the sample area enclosed by the blue dashed line in panel (a). (c) Enlarged view of the coupling side of the SQUID array. (d) Electron micrograph of the DQD showing its electrostatic top gates (Al-light gray) and the plunger gate coupled to the SQUID array (red). (e) Electron micrograph of the transmon SQUID. (f) Circuit diagram schematically displaying the DQD [with its source (S) and drain (D) contact], capacitively coupled to the SQUID array resonator, which in turn is coupled to the transmon. The transmon and the SQUID array are respectively capacitively coupled to a 50 Ω CPW resonator and microwave feedline. Their resonance frequencies can be tuned by using a flux line and a coil schematically shown in the circuit diagram. The color code is consistent with the optical micrographs. loop by a flux line (purple in Fig. 1). We read out the state of We characterize the hybrid circuit by measuring the ampli- the transmon qubit with a 50 Ω coplanar waveguide resonator tude and phase change of the reflection coefficient of a coher- (dark blue in Fig. 1) capacitively coupled to the qubit [6, 35]. ent tone at frequency !p reflected from the multiplexed res- The DQD charge qubit [Fig. 1(d)], schematically indi- onators (the microwave setup is presented in Extended Data cated by the 2 light blue dots in Fig. 1(f), is defined by Fig. 1). The response changes with the potentials applied standard depletion gate technology using Al top gates on a to the gate electrodes forming the DQD and the magnetic GaAs/AlGaAs heterostructure that hosts a two-dimensional flux applied to the transmon. By varying the DQD detun- electron gas (2DEG) [7, 24, 36]. The DQD is tuned to the ing δ and the transmon flux Φtr, each qubit is individually few-electron regime and its excitation energy is given by tuned into resonance with the high-impedance resonator. The p 2 2 coupling strengths measured between the SQUID array res- !DQD = 4tc + δ , with the inter-dot tunnel rate tc and the DQD energy detuning δ. onator and the DQD charge qubit and the transmon qubit are 2gDQD,Sq=2π 66 MHz (at !r,Sq=2π = 4:089 GHz) We use a superconducting high-impedance resonator for ∼ and 2gtr,Sq=2π 451 MHz (at !r,Sq=2π = 5:18 GHz), re- mediating interactions between the transmon and the DQD ∼ [7]. The resonator is composed of an array of 35 SQUIDs spectively, for more details see Methods and Extended Data [Fig. 1(b)] and is capacitively coupled to both transmon and Fig. 3. For the same configuration, we extract the linewidth of the qubits spectroscopically [7, 34] and find δ!DQD=2π DQD charge qubits [see Fig. 1(b,f)]. It is grounded at one ∼ 3 MHz and δ!tr=2π 0:8 MHz. Both subsystems individ- end and terminated in a small island at the other end to which ∼ a single coplanar drive line is capacitively coupled [green in ually are in the strong coupling regime (2g > κ/2 + γ2) Fig. 1(b,c)]. A gate line extends from the island and forms one with a SQUID array resonator linewidth of κ/2π = (κext + κint)=2π (3 + 5) MHz. of the plunger gates of the DQD [in red in Fig. 1(d)] [7, 36]. ∼ The high impedance of the resonator increases the strength of To demonstrate the coherent coupling between the trans- the vacuum fluctuations of electric field, enhancing the cou- mon qubit and the DQD charge qubit, we first character- pling strength of the individual qubits to the resonator (see ize the configuration with the three systems interacting res- Methods for more information).
Details
-
File Typepdf
-
Upload Time-
-
Content LanguagesEnglish
-
Upload UserAnonymous/Not logged-in
-
File Pages9 Page
-
File Size-