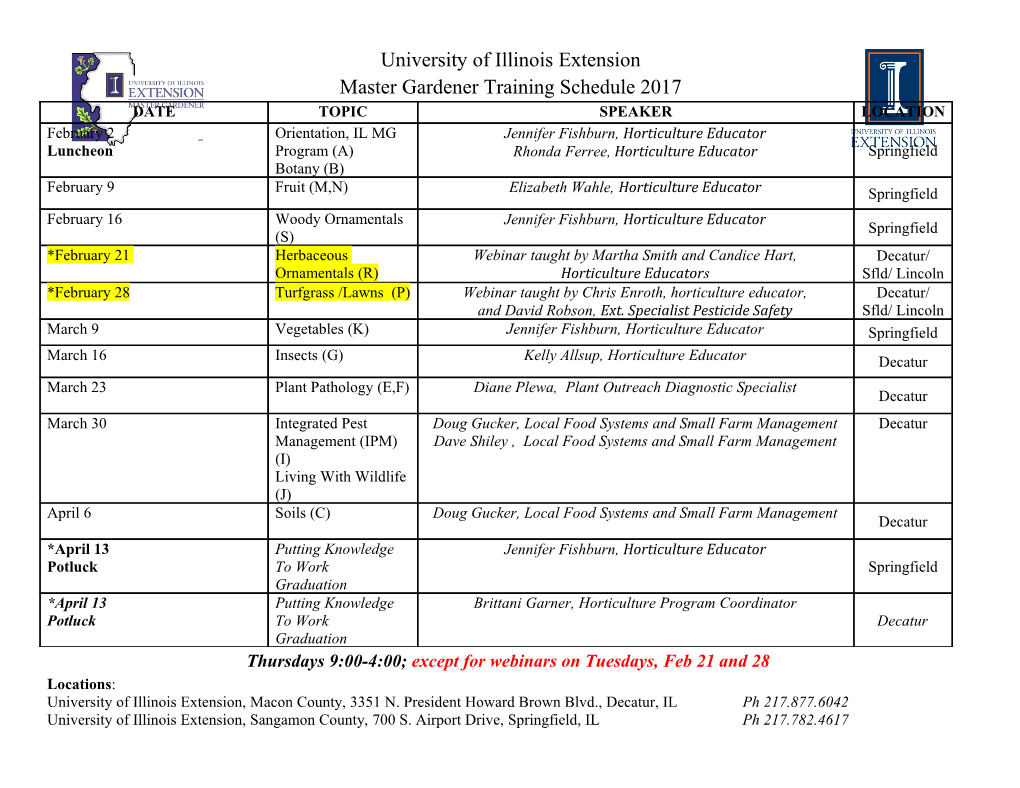
Trans. JSASS Aerospace Tech. Japan Vol. 10, pp. 1-4, 2012 Research Note Vertical Landing Aerodynamics of Reusable Rocket Vehicle 1) 2) 3) 1) 1) By Satoshi NONAKA, Hiroyuki NISHIDA, Hiroyuki KATO, Hiroyuki OGAWA and Yoshifumi INATANI 1) The Institute of Space and Astronautical Science, Japan Aerospace Exploration Agency, Sagamihara, Japan 2) Mechanical Systems Engineering, Tokyo University of Agriculture and Technology, Koganei, Japan 3) Chofu Aerospace Center, Japan Aerospace Exploration Agency, Chofu, Japan (Received November 26th, 2010) The aerodynamic characteristics of a vertical landing rocket are affected by its engine plume in the landing phase. The influences of interaction of the engine plume with the freestream around the vehicle on the aerodynamic characteristics are studied experimentally aiming to realize safe landing of the vertical landing rocket. The aerodynamic forces and surface pressure distributions are measured using a scaled model of a reusable rocket vehicle in low-speed wind tunnels. The flow field around the vehicle model is visualized using the particle image velocimetry (PIV) method. Results show that the aerodynamic characteristics, such as the drag force and pitching moment, are strongly affected by the change in the base pressure distributions and reattachment of a separation flow around the vehicle. Key Words: Counter Jet, Reusable Rocket, Landing Aerodynamics Nomenclature (VTVL) rocket vehicles are a type of reusable space transportation system. In the 1990s, the Delta Clipper 2 CD : drag coefficient, D (ρV∞ S 2) Experimental (DC-X) demonstrated both the key technical feasibility of a VTVL single stage to orbit (SSTO) vehicle 2 Cp : pressure coefficient, − ρ (Psurface P∞ ) ( V∞ S 2) and key operational concepts such as repeatability, safety, D : aerodynamic drag force [N] 1) reliability and inexpensive accessibility to space. The Fj : jet thrust [N] abovementioned feasibility and concepts were 2 fj : momentum flux of jet [kgm/s ] investigated in the framework of the project. The VTVL 2 f∞ : momentum flux of freestream [kgm/s ] rocket concept was studied in Japan. A reusable sounding Mj : Mach number of jet at nozzle exit rocket capable of ballistic flight to an altitude of over 100 Pe : nozzle exit pressure [Pa] km and returning to the launch site has been conceptually Psurface : surface pressure [Pa] proposed by the Japan Aerospace Exploration Agency P0 : thrust chamber pressure [Pa] (JAXA). The reusable vehicle testing (RVT) research S : base area [m2] campaign, which has been in progress since 1999, is a 2 preliminary study that involves the system design of the Snozzle : nozzle exit area [m ] reusable sounding rocket. Furthermore, a small test T0 : thrust chamber temperature [K] vehicle called RVT was built and flight-tested2-5) in the V : freestream velocity [m/s] ∞ research campaign. V : jet velocity (at nozzle exit) [m/s] j To achieve a successful re-entry flight of the reusable α : angle of attack [deg] vehicle, its aerodynamic characteristics must be ρ 3 : freestream density [kg/m ] thoroughly investigated. In the vertical landing phase, the 3 ρj : jet density [kg/m ] main engine is re-ignitioned. The vehicle is decelerated by the main engine thrust and lands gently on the ground. In 1. Introduction such a situation, the main engines eject supersonic plumes that encounter a subsonic outer flow around the vehicle. To develop future space markets, significant progress in The encounter creates a complicated flow structure that the capability of space transportation systems is required involves large-scale vortices. Freestream-counterflow jet to meet the demands of large-scale transportation systems. interactions, where a fluid jet is ejected in front of a body The development of a fully reusable transportation system in supersonic or hypersonic flight conditions, are is required to reduce the transportation cost. A cost investigated in order to understand the mechanism of drag reduction will enable future space activities such as space reduction due to the change in the shock wave position.6-8) tourism and the construction of huge structures like space The engine plume-freestream interaction significantly solar power stations. Vertical takeoff and vertical landing affects the aerodynamic characteristics of the vehicle in 9) © 2012 The Japan Society for Aeronautical and Space Sciences this phase of flight and also causes a problem during safe 1 Trans. JSASS Aerospace Tech. Japan Vol. 10 (2012) landing of the vehicle. The vehicle flight scheme should following parameters are made to correspond to the flight be carefully designed considering the abovementioned conditions: Mach number of the jet at the nozzle exit (Mj aerodynamic problem. However, the = 2.41), ratio of the nozzle exit pressure to the freestream-counterflow jet interaction has not been atmospheric pressure (Pe/Pa = 1.38) and ratio of the 2 investigated in the subsonic region and the mechanism of momentum flux of the jet (fj = ρjv j × Snozzle) to the drag reduction might be different from that in the freestream momentum flux defined by the base area (f∞ = 2 supersonic or hypersonic regions, because the freestream ρ∞ v ∞ ×S); fj/f∞ = 0.77. The momentum flux ratio is shock wave does not appear in the subsonic region. defined by neglecting the pressure term. Owing to the The objective of this study is to experimentally correspondence of these parameters, the ratio of the investigate the aerodynamic effects of the interaction aerodynamic force to the jet thrust force in the flight between a subsonic flow around a vehicle and supersonic condition is simulated in the wind tunnel test. The flight jet from the engine. Experiments on aerodynamic force and wind tunnel test conditions are summarized in Table 1. measurement, surface pressure measurement, and flow Vertical landing rockets need to have the capability of visualization are conducted using the PIV method via a thrust level throttling over a wide range. Therefore, the scale model of RVT in a low-speed wind tunnel. effect of the jet thrust level must be investigated over a range of values. For this reason, the chamber pressure in 2. Test Facilities and Conditions the testing model is controlled to change the thrust level. The chamber pressure P0 is 2.08 MPa in the case of Aerodynamic forces and surface pressures are measured maximum thrust, the freestream velocity in the wind in a Göttingen-type low-speed wind tunnel at JAXA, tunnel V∞ is 26.4 m/s, and the angle of attack is 0 degrees. which has a test section of diameter 1.6 m and length 2.0 The wind tunnel test conditions are summarized in Table m. Furthermore, it has a velocity ranging from 10 to 60 2. In the case of 0, as given in Table 2, the jet is not m/s at 1 atm. It is possible to change the wind tunnel ejected. operation from an open type to a closed type. In the In the experiments performed, the Reynolds number of closed-type operation, the static pressure of the flow is the freestream and the counter jet are approximately 3.5 × varied within the range of 0.1 to 1.0 atm. Measurement of 105 in the critical Reynolds number regime and 5.0 × 106 velocity distributions using the PIV technique is in the supercritical Reynolds number regime, respectively. conducted in another closed circuit wind tunnel at JAXA The Reynolds numbers obtained in the experimental which has a 2.0 m by 2.0 m test section and velocity range conditions are lower than those obtained in the real of 3 to 60 m/s. condition by one order of magnitude. The counter jet Figure 1 shows the RVT vehicle and its 1/12 scaled possesses a turbulent flow in both the experimental and model for wind tunnel tests. The total body length and real conditions. Although the vortex structure in the real base diameter of the actual RVT vehicle are 3.0 and 2.2 m, condition is expected to become smaller than that in the respectively. The wind tunnel model has a high-pressure experimental condition, the comprehensive structure of chamber and nozzle which is placed inside the model to the jet/freestream interaction is dominated by the produce the counter jet. The nozzle throat and exit momentum flux ratio of the freestream against the counter diameters are 2 and 3 mm, respectively. Nitrogen gas is jet. The freestream around the testing model is a transient supplied through flexible tubes. Aerodynamic forces are flow; whereas the flow around the real vehicle is a measured by a six-component internal balance. To turbulent flow. In general, the flow separation point in the evaluate the influence of jet/freestream interactions on the transient flow is very different from that in the turbulent aerodynamic forces, the model surface pressures are flow. However, the slender body used in this study has a measured using differential pressure gauges. Five pressure corner around the base, whose curvature radius is small. ports are located on the model surface, as shown in Fig. Therefore, the flow separation point is fixed at the corner 1(b). The surface of the model is painted with glossy regardless of the flow Reynolds number. As mentioned black paint to reduce laser scattering for flow above, although the flow Reynolds number in our visualization using PIV. experiments is an order of magnitude different from that A two-dimensional PIV system is used in this in the real conditions, the comprehensive flow structure experiment.10) The PIV measurement is performed using around the testing model is qualitatively the same as that two cameras to obtain a wide image of the flowfield around the real vehicle. Therefore, the experimental around the model. Oil particles with diameter of results are expected to include information for research approximately 1 µm are introduced in both the freestream purposes to qualitatively understand the characterization and the jet flow and are visualized using a double pulse of the aerodynamic force acting on the body in relation to Nd: YAG laser.
Details
-
File Typepdf
-
Upload Time-
-
Content LanguagesEnglish
-
Upload UserAnonymous/Not logged-in
-
File Pages4 Page
-
File Size-