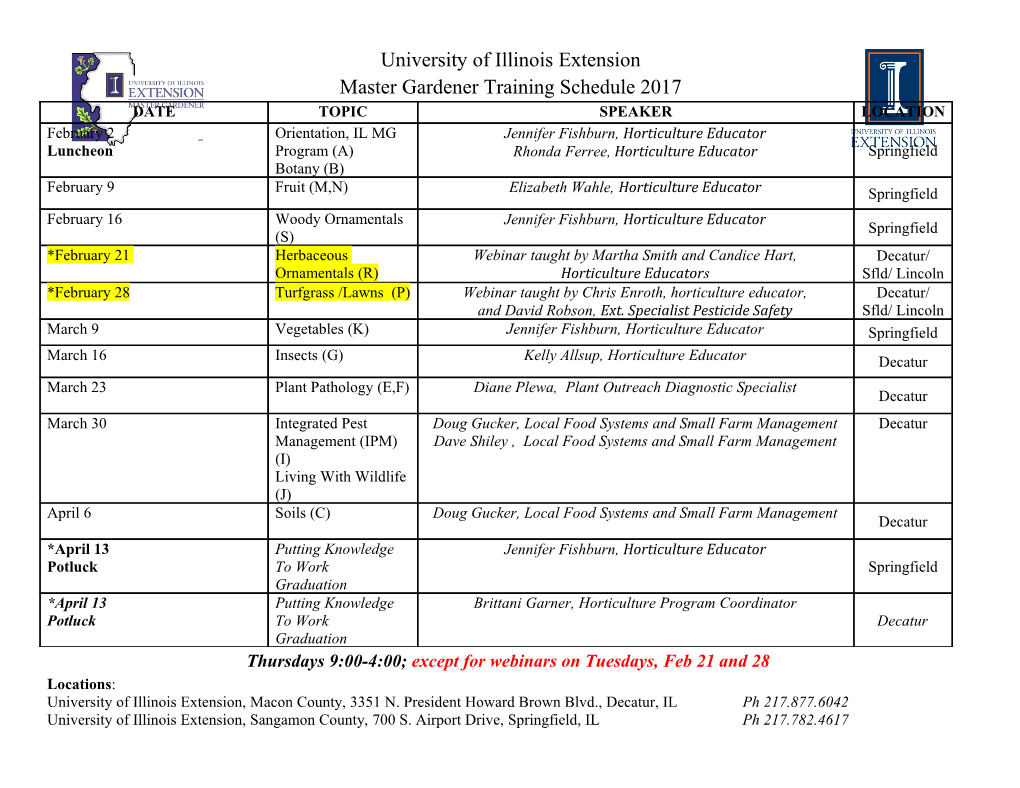
Multi-scale Observations and Modeling of West African Tropical Rainfall Systems: AMMA-Weather Principal Investigators: Chris D. Thorncroft SUNY at Albany Chris Davis NCAR Robert A. Houze University of Washington Richard H. Johnson Colorado State University Steven A. Rutledge Colorado State University Bradley F. Smull University of Washington PROJECT OVERVIEW Executive Summary AMMA-Weather is designed to improve both fundamental understanding and weather prediction in the area of the West African monsoon through deployment of U.S. surface and upper-air observing systems in July and August 2006. These systems will be closely coordinated with International AMMA. The project will focus on the interactions between African easterly waves (AEWs) and embedded Mesoscale Convective Systems (MCSs) including the key role played by microphysics and how this is impacted by aerosol. The pronounced zonal symmetry, ubiquitous synoptic and mesoscale systems combined with the close proximity of the Saharan aerosol make the WAM an ideal natural laboratory in which to carry out these investigations. The observations will provide an important testbed for improving models used for weather and climate prediction in West Africa and the downstream breeding ground for hurricanes in the tropical Atlantic. The international AMMA program consists of scientists from more than 20 countries in Africa, Europe, and the US. Owing to the efforts of European countries, a strong infrastructure is being installed providing a unique opportunity for US participation. Support in excess of twenty million Euros has already been secured by Europeans for AMMA including the 2006 field campaign. Significantly for AMMA-Weather, this will include support for the U.S. NOAA-P3 aircraft, enhancements to the rawinsonde network, European aircraft including dropsondes and aerosol measurements as well as ground-based radar and surface-based measurements (including rainfall and surface fluxes). To address the scale interactions objectives of AMMA-Weather, observations and analysis are required on four scales: synoptic, mesoscale, convective and microscale. We propose to achieve this through a combination of strategically located soundings and specialized radars. We propose to enhance the rawinsonde network to support the analysis of AEWs, the impact of MCSs on the synoptic scale, and the modulations of these effects by the diurnal cycle. The 3D analysis of the kinematic and microphysical structure of the MCSs within the synoptic framework will be afforded by the NCAR S-Pol radar, a C-band Doppler radar (either the NASA/TOGA or MIT radar) and an S-band vertical profiler. In addition, dual-Doppler observations made by a NOAA P-3 turboprop aircraft will be used to extend the dual-Doppler coverage of the ground-based radars and in particular to follow the evolution of vortex structures developing in the stratiform regions of MCSs. These observations will form the basis of a hierarchy of modeling activities ranging from operational modeling, to cloud-system resolving models, to idealized modeling studies. 1. Science Background From a large-scale perspective the West African monsoon (WAM) can be described in terms of the annual march of the ITCZ and its associated regional circulations. On the synoptic and mesoscale, the WAM is comprised of a complex collection of wave patterns, organized weather systems and deep convection (see front page). These include synoptic systems such as African easterly waves (AEWs) (e.g. Reed et al, 1977) and mesoscale convective systems (MCSs) (e.g. Houze and Betts, 1981). AEWs also initiate many of the Atlantic tropical cyclones (TCs) downstream (e.g. Avila and Pasch, 1992) and thus are an important part of the interactions that take place between West Africa and the tropical Atlantic. AMMA-Weather will strive to understand the nature and variability of individual weather systems that comprise the WAM. It will expand upon the earlier studies of this region, in particular, GATE (Houze and Betts, 1981) and COPT81 (Sommeria and Testud 1984, Chauzy et al. 1985, Chong et al. 1987, Roux 1988, Sun and Roux 1988, Chong and Hauser 1989). Those studies were conducted over 20 years ago and therefore did not benefit from today’s advanced observational technologies. They were also carried out with a more limited understanding of tropical dynamics and convection than we now have as a result of TOGA COARE (Godfrey et al. 1998), TRMM (Kummerow et al. 1998), other field campaigns, and advances in analysis of satellite data (Wheeler and Kiladis, 1999) and observational platforms such as portable, polarimetric radars, as well as numerous theoretical studies of tropical wave dynamics and other monsoon systems (Webster 1994, Webster et al. 2001, Thorncroft and Hoskins, 1994a,b, Thorncroft and Blackburn, 1999). AEJ 90oC 50oC θ θe θe θ 60oC 20oC Figure 1.1 Schematic of N-S vertical cross section along the Greenwich Meridian, highlighting the heat low-AEJ-ITCZ system and SAL (shaded yellow) and meridional variations in atmospheric boundary layer θ and θe. Adapted from Parker et al. (2004a). A key feature of the West African monsoon system is the marked meridional contrast in surface properties. Meridional variations in albedo and vegetation between the desert to the north and rainforest to the south impact the surface fluxes and the boundary layer θ and θe distributions (e.g. Nicholson et al 1998; Cook 1999). θ peaks around 25˚N in the Sahara while θe peaks further south in the rainy zone around 10˚N (Fig. 1.1). Deep moist convection in the high θe region and dry convection in the high θ region impact the nature of the large-scale meridional circulations and help establish the African easterly jet (AEJ, Thorncroft and Blackburn, 1999). As highlighted in the recent observations made during the JET2000 aircraft campaign (Thorncroft et al, 2003, Parker et al, 2004), the baroclinic region between is characterized by two coherent layers: the humid monsoon layer affected by the land surface on diurnal timescales and above this the Saharan air layer (SAL) characterized by low humidity and high aerosol content (shaded yellow in Fig. 1.1). The JET2000 observations indicate that the boundary layer air in this baroclinic zone is more buoyant than the higher θe region south of this, consistent with the presence of a capping inversion provided by the SAL. The presence of the SAL in the baroclinic zone also highlights the potentially important role of aerosol in influencing the thermodynamics and microphysics in this region. Given these contrasts we expect marked contrasts in the nature of convection and MCSs across the baroclinic region – AMMA provides us with an opportunity to investigate these contrasts. The AEJ-environment supports the growth of MCSs and AEWs and in general both types of system co-exist and dominate the WAM (Fig. 1.2 and front page). It is important that we have a good description and understanding of the AEWs and MCSs, their interactions with each other and the large-scale environment (see Fig. 1.2). A complete description of these interactions must also include a consideration of the nature of the convection and how this is influenced by the both the large-scale environment (Fig.1.1) and microphysical processes including the impact or aerosol. Although these scale interactions are central to the WAM, we lack a good description and understanding of how they operate and combine to produce the observed WAM and its variability. The extent to which GCMs used for weather and climate prediction are able to represent these scale interactions, explicitly or through parameterizations requires investigation. We are hindered in carrying out such investigations due to lack of appropriate multi-scale observations. SAL AEWs TC MCSs Figure 1.2 Key weather systems in the West African and Tropical Atlantic regions. Solid line represents a streamline at the level of the AEJ around 600hPa. Grey shading represents peak rainfall and yellow shading indicates the location of the SAL. 1.1 African easterly waves African easterly waves (AEWs) are synoptic-scale weather systems that characterize the WAM and downstream tropical Atlantic during boreal summer. They have a zonal wavelength of 2000-3000 km and propagate westwards along the mid-tropospheric African easterly jet (AEJ) located around 15oN and 600 hPa (Fig. 1.1). These are important systems in the WAM region due to their association with daily rainfall (e.g. Reed et al, 1977), because they can serve as precursors for tropical cyclones (e.g. Avila and Pasch, 1992) and because they influence the spatial and temporal variability of the dry and aerosol laden Saharan air layer (SAL). Despite their importance, fundamental gaps exist in our knowledge and understanding of the evolution of AEWs as they move across Africa and the Atlantic; especially how the AEW dynamics interacts with MCSs and convection. AEWs are usually viewed as developing via a mixed barotropic-baroclinic instability mechanism in association with perturbations of the meridional gradients of potential vorticity (PV) in the core of the AEJ and meridional gradients of low-level potential temperature (θ) polewards of the AEJ (Burpee, 1972, Thorncroft and Hoskins, 1994a). Consistent with this, analyses indicate that AEWs tend to be characterized by peak amplitudes in two locations; one in the vicinity of the mid-level PV gradients in the rainy zone and one in the vicinity of the low- level θ gradients polewards of this where it rains much less (Reed et al, 1977, Pytharoulis and Thorncroft, 1999). Composite analyses of AEWs (Fig. 1.3, Reed et al, 1977, Burpee, 1972) still dominate our perception of an AEW. While such studies provide useful information about the mean structure of AEWs and supporting evidence for the role played by barotropic-baroclinic instability, by exhibiting characteristic horizontal and vertical tilts, the statistical averaging involved in such studies provides us with an ‘out-of-focus’ view of AEWs.
Details
-
File Typepdf
-
Upload Time-
-
Content LanguagesEnglish
-
Upload UserAnonymous/Not logged-in
-
File Pages39 Page
-
File Size-