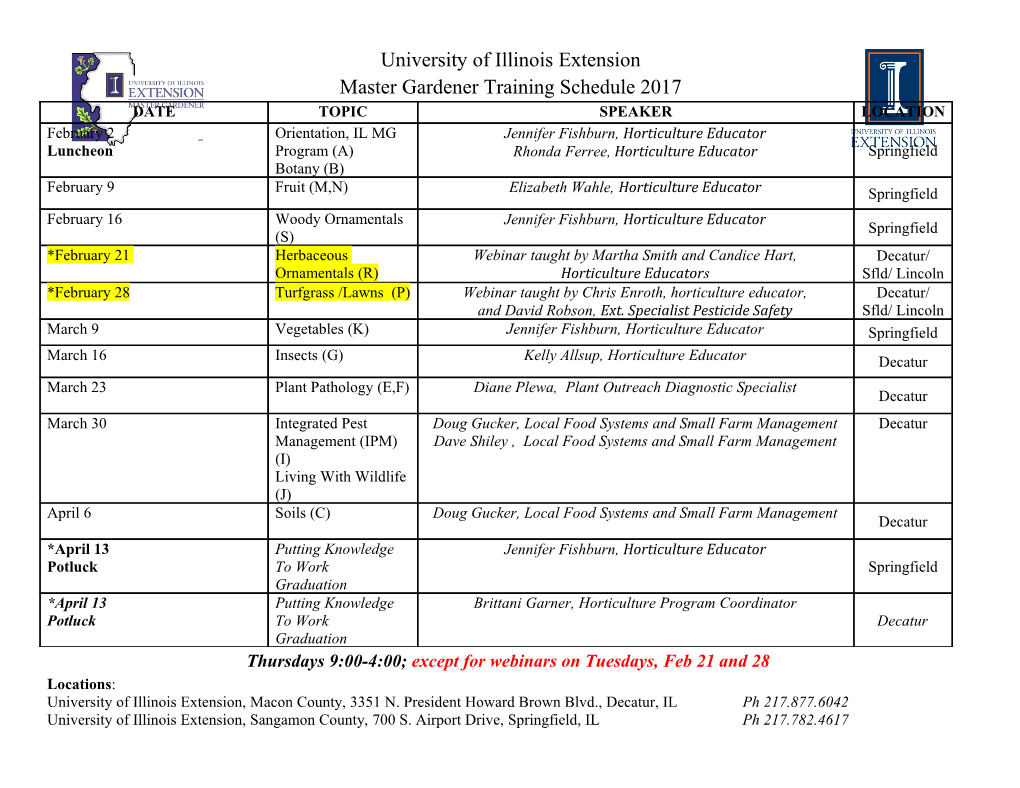
Chapter 13 Membrane-Bound Dehydrogenases of Acetic Acid Bacteria Osao Adachi and Toshiharu Yakushi Abstract One of the major key features of acetic acid bacteria is their strong oxidation ability of alcohols and sugars, resulting in quantitative production of oxidized compounds. Respiratory chains consisting of ubiquinone, terminal ubiquinol oxidase, and several primary membrane-bound dehydrogenases are responsible for this unique ability. Here, we describe recent progress in the under- standing of enzymatic and molecular properties and biogenesis of the membrane- bound dehydrogenases, such as pyrroloquinoline quinone-dependent alcohol dehydrogenase–cytochrome complex, and recent findings on new membrane- bound dehydrogenases. Quinate oxidation by quinate dehydrogenase (QDH) of acetic acid bacteria is a key agent in the vitro shikimate production process composed of the membranes containing QDH and 3-dehydroquinate dehydratase and NADP+-dependent shikimate dehydrogenase. The addition of a catalytic amount of NADP+ and an NADPH-regeneration system drive the process forward to produce shikimate with almost 100 % yield. The pentose oxidation respiratory chain produces 4-keto-D-arabonate or 4-keto-D-ribonate, depending on the substrate. Novel three different membrane-bound enzymes are indicated: D-aldopentose 4-dehydrogenase, 4-keto-D-aldopentose 1-dehydrogenase, and D-pentonate 4-dehydrogenase. Keywords Flavoprotein • Molybdoprotein • Quinoportein • Quinate • Respiratory chain • Shikimate • 4-Keto-D-aldopentonate • D-Aldopentonate O. Adachi (*) Department of Biological Chemistry, Faculty of Agriculture, Yamaguchi University, Yoshida 1677-1, Yamaguchi 753-8515, Japan e-mail: [email protected] T. Yakushi (*) Department of Biological Chemistry, Faculty of Agriculture, Yamaguchi University, Yoshida 1677-1, Yamaguchi 753-8515, Japan Research Center for Thermotolerant Microbial Resources, Yamaguchi University, Yoshida 1677-1, Yamaguchi 753-8515, Japan e-mail: [email protected] © Springer Japan 2016 273 K. Matsushita et al. (eds.), Acetic Acid Bacteria, DOI 10.1007/978-4-431-55933-7_13 274 O. Adachi and T. Yakushi Fig. 13.1 Substance oxidation respiratory chains of acetic acid bacteria. Many kinds of membrane-bound dehydrogenases oxidize the cognate substrates with coupling to reduction of ubiquinone (UQ). The reduced ubiquinone (UQH2) is then directly reoxidized by the terminal ubuquinol oxidase (Cyt. bo3). The colors of Enz 1, Enz 2, and Enz 3 are related to those in Fig. 13.7. DEC, putative 2,5-diketo-D-gluconate decarboxylase; other abbreviations are referred to the text 13.1 Introduction Incomplete oxidation with acetic acid bacteria is carried out through oxidation reactions by membrane-bound dehydrogenases located on the periplasmic surface of the cytoplasmic membrane. These dehydrogenases reduce membranous ubiqui- none (UQ) coupled with the substrate oxidation. Reduced form of ubiquinone (UQH2) is then directly re-oxidized by the terminal ubiquinol oxidases. Thus, several kinds of the dehydrogenases function as the primary dehydrogenase in substance-oxidizing respiratory chains (Matsushita et al. 1994) (Fig. 13.1). For example, ethanol- and glucose-oxidizing respiratory chains carry out incomplete oxidations of ethanol and D-glucose, respectively. Although some enzymes are poorly examined in their physiological electron acceptor, all the membrane- bound dehydrogenases mentioned in this chapter are thought to react with mem- branous ubiquinone (Table 13.1). We briefly review “conventional” membrane- bound dehydrogenases of acetic acid bacteria in the first part. The second part explains new membrane-bound dehydrogenases found recently and their applications. Table 13.1 Membrane-bound dehydrogenases of acetic acid bacteria 13 Membrane-Bound Dehydrogenases of Acetic Acid Bacteria 275 Category Enzyme Substrate Product Subunit Prosthetic Prosthetic Electron References composition group 1a group 2a acceptorb Quinoprotein– ADH Ethanol Acetaldehyde αβγ or αβ PQQ 4 Heme C UQ Yakushi and cytochrome complex EC1.1.5.5 Matsushita (2010) Molybdoprotein– ALDH Acetaldehyde Acetic acid αβγ MCD 2 [2Fe-2S] À Thurner cytochrome complex EC1.2.99.3 3 Heme C et al. (1997) Heme B Flavoprotein– GADH D-Gluconate 2-Keto- αβγ FAD 3 Heme C UQ Shinagawa cytochrome complex EC1.1.99.3 D-gluconate et al. (1984) 2KGDH 2-Keto- 2,5-Diketo- αβγ FAD 3 Heme C À Shinagawa EC1.1.99.4 gluconate D-gluconate et al. (1981) FDH D-Fructose 5-Keto-D-fructose αβγ FAD 3 Heme C À Ameyama EC1.1.99.11 et al. (1981a) SLDH D-Sorbitol L-Sorbose αβγ FAD 3 Heme C À Shinagawa EC1.1.99.21 et al. (1982) Membrane-bound GDH D-Glucose Glucono-δ-lactone α PQQ À UQ Ameyama quinoprotein EC1.1.5.2 et al. (1981b) GLDH Polyols Ketones αβ PQQ À UQ Matsushita EC1.1.99.22 et al. (2003) QDH Quinate 3-Dehydroquinate α PQQ À UQ Vangnai EC1.1.5.8 et al. (2004) IDH myo-Inositol 2-Keto- α PQQ ÀÀHolscher€ myo-inositol et al. (2007) (continued) Table 13.1 (continued) 276 O. Adachi and T. Yakushi Others SDH L-Sorbose L-Sorbosone α FAD À UQ Sugisawa EC1.1.99.12 et al. (1991) SNDH L-Sorbosone 2-Keto-L-gulonate α ÀÀÀShinjoh et al. (1995) aProsthetic groups 1 and 2 that are involved in substrate oxidation and that are involved in intramolecular electron transfer, respectively bPhysiological electron acceptor: those for all the dehydrogenases are assumed to be ubiquinone, but UQ (ubiquinone) was placed in the table based on experimental data 13 Membrane-Bound Dehydrogenases of Acetic Acid Bacteria 277 13.2 Fermentation and Membrane-Bound Dehydrogenases Acetic acid fermentation is carried out by sequential oxidations of ethanol to acetic acid via acetaldehyde. The membrane-bound, pyrroloquinoline quinone (PQQ)- dependent alcohol dehydrogenase (ADH) and membrane-bound aldehyde dehydro- genase (ALDH) constitute the ethanol-oxidizing respiratory chain on the outer surface of the cytoplasmic membrane of acetic acid bacteria: Komagataeibacter, Acetobacter, and Gluconobacter (Thurner et al. 1997; Yakushi and Matsushita 2010; Adachi et al. 1980). Unfortunately, it was long misunderstood that these oxidation reactions are mediated by cytoplasmic NAD+- or NADP+-dependent dehydrogenases. However, explanations for acetic acid fermentation were revised in the textbook as the current understanding in 2003 (Madigan et al. 2003). Gluconate fermentation is carried out by a single oxidation of D-glucose to produce glucono-δ-lactone, which is converted to D-gluconate spontaneously or with membrane-bound gluconolactonase. 2-Ketogluconate and 5-ketogluconate fermentations are carried out by a single oxidation in position C2 or C5 of D-gluconate, respectively. 2-Ketogluconate (2KGA) is further oxidized at position C5 to produce 2,5-diketogluconate. 13.3 Membrane-Bound Dehydrogenases: An Overview We take a quick look on the series of membrane-bound dehydrogenase of acetic acid bacteria. We try to categorize them into five groups, that is, quinoprotein– cytochrome complex, molybdoprotein-cytochrome complex, flavoprotein– cytochrome complex, membrane-bound quinoprotein, and others, in terms of their primary structure. 13.4 Quinoprotein–Cytochrome Complex ADH is an ethanol:ubiquinone oxidoreductase that catalyzes oxidation of ethanol to produce acetaldehyde coupling with reduction of membranous ubiquinone (EC 1.1.5.5). Because the reaction by ADH is carried out in the periplasmic side of the membrane, ADH itself does not produce a proton gradient across the membrane. The enzymatic reaction of ADH was clearly demonstrated by the reconstitution experiments of ethanol-oxidizing respiratory chain in the proteoliposomes consisting of ADH, ubiquinone, and the terminal ubiquinol oxi- dase (Matsushita et al. 1992). ADH is a peripheral membrane protein localizing on the periplasmic surface of the cytoplasmic membrane without a predictable trans- membrane segment. Depending on the bacterial species, ADH consists of at least two subunits: the dehydrogenase subunit, having one molecule each of PQQ and 278 O. Adachi and T. Yakushi Quinoprotein Molybdoprotein Flavoprotein Acetaldehyde Acetic D- 2-Keto-D- acid Gluconate gluconate Ethanol Acetaldehyde MCD FAD PQQ III I I I II III II c Fe-S III II Cyt. c Cyt. c Cyt. c Periplasm Cytoplasmic QQH QQH2 2 QQH2 membrane Cytoplasm Fig. 13.2 Cartoon for three types of dehydrogenase–cytochrome complex: predicted structures of ADH, ALDH, and GADH. Although the dehydrogenase (large) and small subunits (shown as I and III, respectively) are not similar to one another, the cytochrome subunit (II) shows significant homology. See Chap. 7 by Matsushita and Matsutani for detailed phylogenetic analysis and discussion heme C, and a cytochrome subunit having three hemes C (Fig. 13.2) (Adachi et al. 1978; Tayama et al. 1989). The cytochrome subunit is responsible for reduction of ubiquinone (Matsushita et al. 1996). In the Acetobacter and Gluconobacter enzymes, an additional small subunit seemingly having no pros- thetic group associates with the ADH complex (Kondo et al. 1995). The adhAB genes for the large and cytochrome subunits constitute an operon, but the gene for the small subunit is mono-cistronic, apart from the adhAB operon. After protein synthesis on the ribosome in the cytosol, all three subunits are transported to the periplasmic space via the Sec protein translocation system, which is a general protein secretion system in gram-negative bacteria. In the periplasmic space, PQQ and heme C are incorporated into the transported proteins along with the protein folding, because the Sec system translocates proteins
Details
-
File Typepdf
-
Upload Time-
-
Content LanguagesEnglish
-
Upload UserAnonymous/Not logged-in
-
File Pages26 Page
-
File Size-