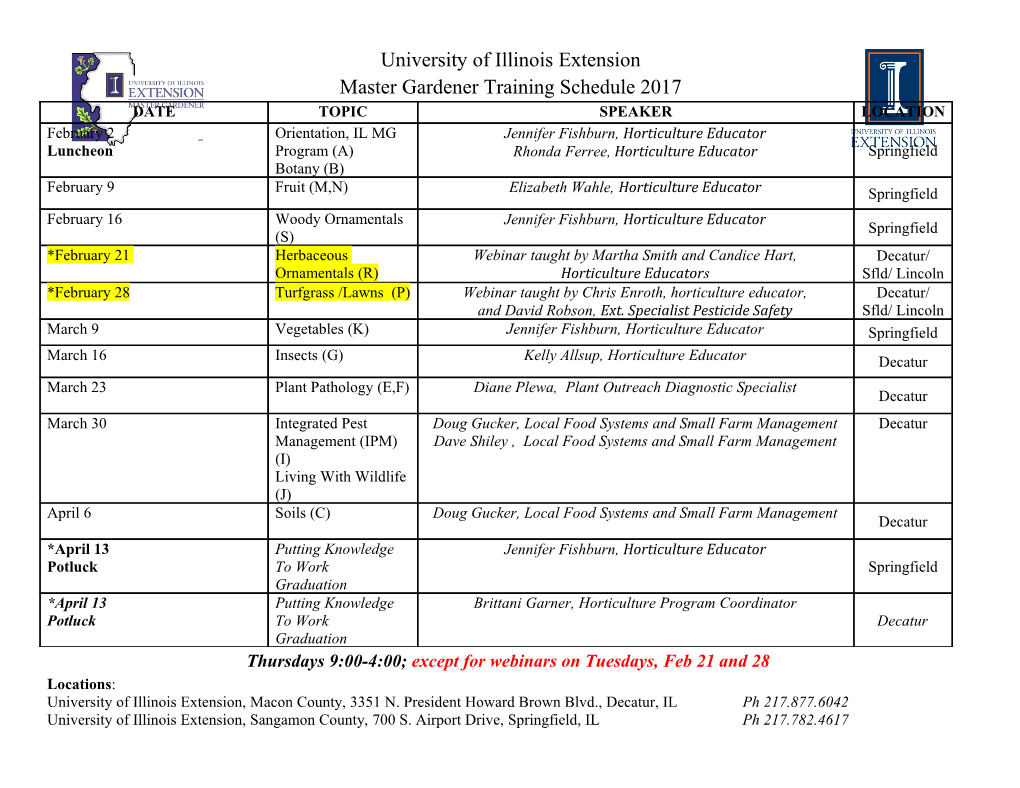
materials Article From a Basic Microalga and an Acetic Acid Bacterium Cellulose Producer to a Living Symbiotic Biofilm Vítor Nóbrega 1, Marisa Faria 1,2, Antera Quintana 3, Manfred Kaufmann 4,5 , Artur Ferreira 6 and Nereida Cordeiro 1,5,* 1 LB3, Faculty of Science and Engineering, University of Madeira, 9000-390 Funchal, Portugal 2 Oceanic Observatory of Madeira (OOM), ARDITI, Madeira Tecnopolo, 9020-105 Funchal, Portugal 3 Banco Español de Algas, Instituto de Oceanografía y Cambio Global (IOCAG), Universidad de Las Palmas de Gran Canaria, 35214 Telde, Spain 4 Marine Biology Station of Funchal, Faculty of Life Sciences, University of Madeira, 9000-107 Funchal, Portugal 5 CIIMAR-Interdisciplinary Centre of Marine and Environmental Research, University of Porto, 4450-208 Matosinhos, Portugal 6 CICECO, Aveiro Institute of Materials, University of Aveiro, 3810-193 Aveiro, Portugal * Correspondence: ncordeiro@staff.uma.pt Received: 10 June 2019; Accepted: 10 July 2019; Published: 15 July 2019 Abstract: Bacterial cellulose (BC) has recently been the subject of a considerable amount of research, not only for its environmentally friendly biosynthesis, but also for its high potential in areas such as biomedicine or biomaterials. A symbiotic relationship between a photosynthetic microalga, Chlamydomonas debaryana, and a cellulose producer bacterium, Komagataeibacter saccharivorans, was established in order to obtain a viable and active biofilm. The effect of the growth media composition ratio on the produced living material was investigated, as well as the microalgae biomass quantity, temperature, and incubation time. The optimal temperature for higher symbiotic biofilm production was 30 ◦C with an incubation period of 14 days. The high microalgae presence, 0.75% w/v, and 60:40 HS:BG-11 medium (v/v) induced a biofilm microalgae incorporation rate of 85%. The obtained results report, for the first time, a successful symbiotic interaction developed in situ between an alkaline photosynthetic microalga and an acetic acid bacterium. These results are promising and open a new window to BC living biofilm applications in medical fields that have not yet been explored. Keywords: bacterial cellulose; microalgae; living biofilm; Chlamydomonas debaryana; Komagataeibacter saccharivorans; symbioses 1. Introduction Bacterial cellulose (BC), an exopolymer of Gluconacetobacter sp. bacteria, a subgenus of Acetobacter, has many unique properties such as a sophisticated 3D porous network, long fibre, high crystallinity, high water absorption, and retention capacity [1–3]. The BC chemical structure is composed by (1 4)-D-anhydroglucopyranose chains bounded through β-glycosidic linkages. BC’s geometry is ! determined by the intra-molecular and inter-molecular hydrogen-bonding network, hydrophobic and van der Waals interactions, and forms parallel chains (Cellulose Type I) [2]. Despite similarities to other nanocellulose types like cellulose nanofibers (CNF) or cellulose nanocrystals (CNC), BC nanofibrils have unique properties such as more crystallinity, a higher degree of polymerization, and the ability to form an ultrafine web structure that makes it difficult to disperse [4]. Furthermore, BC also has a good mechanical strength and allows for a relatively easy shaping control, e.g., through a container´s shape Materials 2019, 12, 2275; doi:10.3390/ma12142275 www.mdpi.com/journal/materials Materials 2019, 12, 2275 2 of 13 where the bacteria are cultured [5]. This biopolymer is generally produced in a static media culture and forms a gelatinous membrane on the interface between air and the culture (aerobic microorganisms) [6]. Moreover, an important asset of BC is its excellent biocompatibility. Many studies have described the successful interactions between BC and other cell types or microorganisms. One example is described by Hu et al. who reported that there was a positive biocompatibility with human osteoblasts, where the osteoblasts were able to grow and rapidly attach themselves in the BC [7]. Furthermore, Czaja et al. reported a new wound healing system based on BC, which clinically investigated the treatment of skin burns on humans [8]. In this research, the wound healing effects of never-dried BC membranes were compared with conventional gauze wound dressings (controls). These studies were proceeded by in vivo tests conducted on animal models and showed that BC membranes were fully biocompatible and do successfully protect burn wounds by preventing excessive external fluid loss, which stimulates a more rapid healing process. In order to create the optimal conditions for the mutual growth and a sustainable symbiotic process (association between two or more organisms), some changes must be made to each component of this process, for example to generate an environment that can endure two or more different metabolisms and their conditions [9,10]. One example of well-studied mutuality relationships are lichens, which are commonly known as a symbiotic association of a fungus and a partner containing chlorophylls, either cyanobacteria, green algae, or both [11]. Chlorophyll enriched partners provide photosynthetically fixed carbon as the source of energy for the system, while fungus promotes a suitable substrate for both. However, some literature also reports that lichens produce antibacterial and antifungal compounds [12]. Moreover, recently, many research reports suggest that there is a symbiosis phenomenon between lichens and microorganisms, such as bacteria [13]. These observations unlock a whole new range of possibilities by showing that there could be a conceivable mutual relationship between photosynthetic organisms and microorganisms like bacteria. In fact, a few cases have already been reported. One example is the mutualistic relationship between Chlorella vulgaris (photosynthetic green microalgae) and a symbiotic bacterium, Pseudomonas sp. [14]. Guo and Tong reported different interactions between algae and bacteria when different incubation conditions were applied. Moreover, adaptability is a major factor that contributes to the establishment of a symbiotic relationship. Das et al. reported a symbiosis between Acetobacter aceti and Chlamydomonas reinhardtii were cultured simultaneously [15]. The symbiotic conditions were described as acidic (pH below 6.5), with a 70:30 ratio between the bacteria medium (glucose and yeast) and the microalgae medium (tris-acetate-phosphate), with an optimal temperature between 28 and 30 ◦C. In addition, a symbiotic growth by an acetic acid bacterium and an acid microalga has already been reported [15,16]. However, literature is still lacking on a successful high pH symbiosis achievement. An alkaline association could enable this kind of bacteria to produce a 3D network BC for incorporation of alkaline microalgae such as Chlamydomonas debaryana (a model microalga), and thus, this could define whole new horizons in biomedical treatments and technology. Furthermore, microalgae are also able to use light energy and convert it throughout several membrane protein complexes into electrochemical energy, which is then used to assimilate CO2. This process releases oxygen, an essential molecule for energy production in aerobic organisms such as Komagataeibacter saccharivorans [17]. The aim of this study was to obtain, for the first time, a biofilm composed by C. debaryana cells (basic microalgae) and a BC 3D network produced by K. saccharivorans (acid bacterium), considering its biocompatible and high-water absorption properties. The optimal composition of the culture media which supports symbiotic growth of the living biofilm, the viability of the integrated microalgae, variations on medium acidity and incorporation ratios were then studied. The obtained biofilm was characterized morphologically and structurally through ATR-FTIR, SEM, and fluorescence microscopy methods. Materials 2019, 12, 2275 3 of 13 2. Results and Discussion 2.1. Growth Symbiotic Media Optimization 2.1.1. Carbon Source The production of biomaterials by microorganisms is a process that requires a huge amount of energy, however, its production also has many different functions and benefits. For example, BC has many functions to bacteria, such as the acquisition of oxygen, the prevention of ultraviolet radiation damage, the enhancement of antibiotic resistance, and the retention of moisture [18,19]. Furthemore, BC production process of Gluconacetobacter strains is already well documented and indicates that glucose is the main precursor for cellulose synthesis [20]. Thus, the compounds capable of being transformed into glucose are theoretically viable carbon sources for cellulose producing bacteria. However, an efficient BC production is highly dependent on the carbon source selection [19]. Bacteria can use several sugars for metabolic purposes through distinct pathways. This ability induces the production of different metabolic products that are extruded from bacteria and influences the medium pH value [21]. Previous pilot studies have shown that the acidity of K. saccharivorans culture media was in a hostile environment for C. debaryana, which consequently resulted in an inhibition of the population growth or in a significant decrease of the initial cell population. Therefore, a reformulation of the HS culture medium was necessary for the microalgae’s survival. Based on this, different carbon sources in the culture media were studied. Table1 displays the culture media’s final pH values for the three concentrations of the three different carbon sources. The results show that replacing
Details
-
File Typepdf
-
Upload Time-
-
Content LanguagesEnglish
-
Upload UserAnonymous/Not logged-in
-
File Pages13 Page
-
File Size-