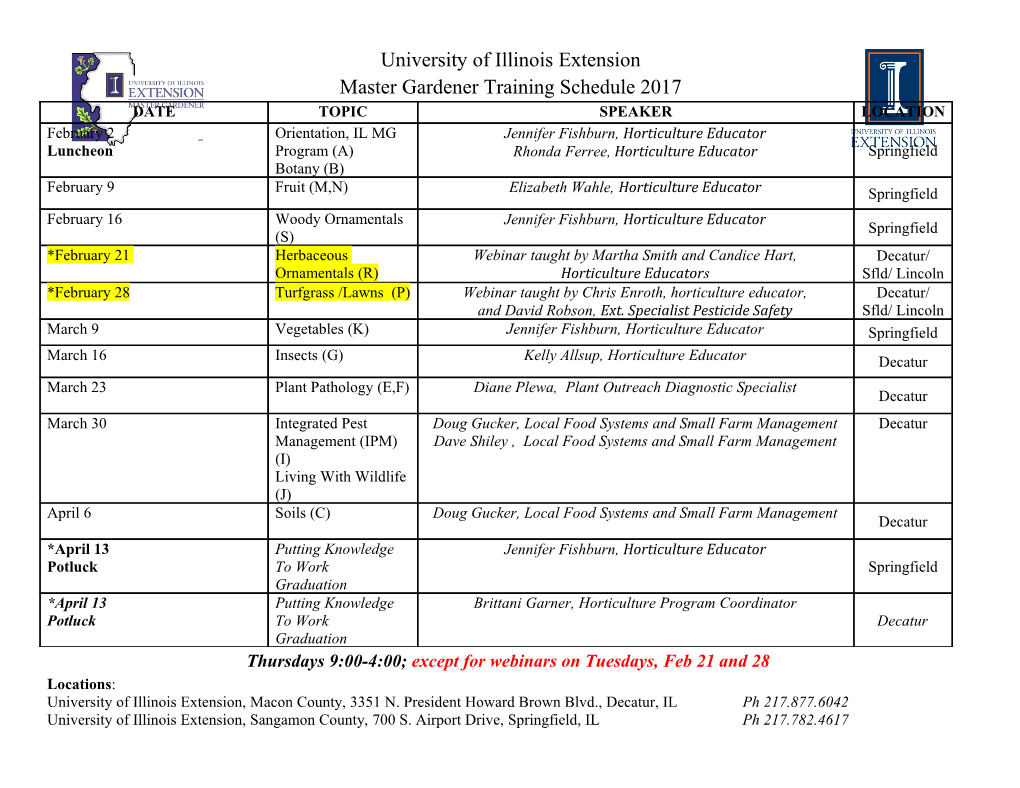
UNDERSTANDING THE INTERACTION BETWEEN GRAIN BOUNDARIES AND PRECIPITATES IN Ni-Al USING MOLECULAR DYNAMICS by RACHEL L. MORRISON Submitted in partial fulfillment of the requirements For the degree of Master of Science Thesis Adviser: Dr. Jennifer L.W. Carter Department of Materials Science and Engineering CASE WESTERN RESERVE UNIVERSITY August, 2018 Understanding the Interaction between Grain Boundaries and Precipitates in Ni-Al Using Molecular Dynamics Case Western Reserve University Case School of Graduate Studies We hereby approve the thesis1 of RACHEL L. MORRISON for the degree of Master of Science on June 26, 2018 Dr. Jennifer L.W. Carter Committee Chair, Adviser Department of Materials Science and Engineering Dr. John J. Lewandowski Committee Member Department of Materials Science and Engineering Dr. David H. Matthiesen Committee Member Department of Materials Science and Engineering Dr. Saryu J. Fensin Secondary Adviser Los Alamos National Laboratory 1We certify that written approval has been obtained for any proprietary material contained therein. Dedicated to Joe Ledger, who believed in me during those moments when I did not believe in myself. Table of Contents List of Tables vi List of Figures vii Acknowledgements xiv List of Abbreviations xv Abstract xvi Abstract xvi Chapter 1. Introduction1 Chapter 2. Background2 Nickel Superalloys2 Grain Boundaries7 Molecular Dynamics 11 Molecular Dynamics and Interfaces 19 Chapter 3. Methodology 22 Simulation Resources 22 S11 Boundary System 24 g0 Precipitate 29 Chapter 4. Results 35 S11 Boundary Structure 35 System Relaxation 36 S11 Deformation Behavior 41 iv g/g0 Deformation Behavior 43 S11/g0 Deformation Behavior 44 Chapter 5. Discussion 57 S11 Behavior 57 g/g0 Deformation 58 g0 Formation 59 g0 Strain Fields 60 g0 Stress Fields 60 Orientation Study 61 Size Study 62 Interaction Study 63 Chapter 6. Conclusions 65 Chapter 7. Suggested Future Research 66 Grain Boundary Morphology 66 Grain Boundary Configuration 66 Appendix A. 67 Appendix. References 71 v List of Tables 3.1 The elastic constants and standard Gibbs free energy as calculated by the Mishin potential1. 23 3.2 An overview of the grain boundary creation steps. The x, y, and z boundaries conditions are listed as periodic (p) or fixed (f). 29 vi List of Figures 2.1 SEM micrograph of the superalloy Rene´ 104 with a deposited oxide grid and speckle pattern. Grain boundary sliding is observed along the cracked boundary, highlighted by the white circles. HRSEM backscatter inset image showing the two 0 primary phases, FCC Ni g channels and L12 Ni3Al cuboidal g precipitates. Micrographs taken from Carter2.3 2.2 Grain boundaries are conventionally defined by a rotation axis (o = [hokolo]), a rotation angle (q), and a boundary plane (nˆ = 3 (hnAknAlnA)). Figure recreated from Lejcek .8 2.3 A grain boundary between two grains that are described with a S5 coincidence site lattice. Every 5th atom is colored white to represent those lattice sites that overlap within the two grains3. 10 2.4 The center (gray) simulation cell is surrounded by images of itself (white), and the equivalent atoms in each cell move in tandem, as shown by the arrows. The atoms within the simulation cell are allowed to interact with the atoms in the periodic cells, and atoms can travel freely across the simulation cell/periodic cell boundary. 15 3.1 Each simulation contains two grains (G1 and G2) separated by a grain boundary (gray line). A g0 precipitate with an orientation (tilt, square, and inline) and size (small, large) is inserted onto the boundary, coherent with G1. 31 vii 3.2 The system is bisected by a grain boundary (light gray plane), and a g0 is inserted in the center of the GB (orange cube). The y boundaries have fixed regions (dark gray) in order to apply a velocity that will drive shear deformation (arrow). The system is cut down in the x and z directions to allow the g0 to interact with its periodic images, as shown partially by the dotted lines. 33 4.1 The kite structure for the S11 boundary as modeled by (left) MD and (right) TEM4 looking down the [110] tilt axis. 35 4.2 The temperature as a function of time during the relaxation of an initialized MD system. The temperature increases to a setpoint, and then reaches a steady state regime. Once the system has reached steady state, further experiments can be run. 36 4.3 The stress per atom averaged in rectangular regions perpendicular to the grain boundary normal. The three principal stress directions, sxx, syy, and szz are shown. 37 4.4 The Gibbs free energy of nucleation for a sphere of radius r and cube of edge length 2r. The energy is calculated based off values from the Mishin potential1. 38 4.5 Strain fields caused by the g0 precipitates prior to deformation. The strain is calculated by the displacement of atoms before and after relaxation, normalized to one unit of slip for nickel. viii Images are a x/z slice of the system. The position of the grain boundary is indicated by the gray line. 39 4.6 Stress fields caused by the g0 precipitates prior to deformation. Images are a x/z slice of the system. 40 4.7 The stress vs. strain of a (left) S11 GB system and (right) g/g0 system without a grain boundary. Both systems experience a stress drop after deformation (GBS or dislocation formation respectively). 41 4.8 The centrosymmetry, excess volume, and normalized displacement of atoms averaged in bins along the y axis of the (left) S11 and (right) g/g0 systems before and after shear deformation. 42 4.9 Snapshots of the S11 GB system during sliding. The (left) initial structure, (center) an intermediate structure, and (right) the disordered boundary after sliding. The atoms are colored according to centrosymmetry; dark blue atoms have a perfect FCC environment and all other colors deviate from an FCC environment. 43 4.10 A snapshot of the g/g0 system under shear deformation. The g0 is colored white, and the remaining atoms are colored according to centrosymmetry; dark blue atoms have a perfect FCC environment and all other colors deviate from an FCC environment. 44 ix 4.11 (left) The stress vs. strain of the S11 and small g0 tilt, square, and inline systems after shear deformation. (right) The displacement of atoms averaged in bins along the y axis of the S11 and small g0 tilt, square, and inline systems after deformation. 45 4.12 The maximum stress of the small tilt, small square, small inline, and S11 systems prior to deformation, measured from the stress strain curves. 46 4.13 The width of the centrosymmetry curves before and after deformation for the small tilt, small square, small inline, and S11 systems, which represents the extent to which the system disorder is localized to the grain boundary (narrower = more localized, wider = less localized). Centrosymmetry curves are shown in AppendixA. 47 4.14 An x/y plane of the small tilt g0 configuration before (left and center) and after (right) GBS. The g0 is coherent with the right grain. Atoms that have an FCC environment are colored dark blue (nickel grains) or white (g0), and all other atoms are colored according to centrosymmetry. 47 4.15 An x/y plane of the small square g0 configuration before (left and center) and after (right) GBS. The g0 is coherent with the right grain. Atoms that have an FCC environment are colored dark blue (nickel grains) or white (g0), and all other atoms are colored according to centrosymmetry. 48 x 4.16 An x/y plane of the small inline g0 configuration before (left and center) and after (right) GBS. The g0 is coherent with the right grain. Atoms that have an FCC environment are colored dark blue (nickel grains) or white (g0), and all other atoms are colored according to centrosymmetry. 48 4.17 (left) The stress vs. strain of the small vs. large g0 tilt, square, and inline systems after shear deformation. (right) The displacement of atoms averaged in bins along the y axis of the small and large g0 tilt, square, and inline systems after shear deformation. 50 4.18 The maximum stress of the small and large tilt, square, inline systems, and the S11 system prior to deformation, measured from the stress strain curves. 51 4.19 The width of the centrosymmetry curves before and after deformation for the small and large tilt, square, inline systems, and the S11 system, which represents the extent to which the system disorder is localized to the grain boundary (narrower = more localized, wider = less localized). Centrosymmetry curves are shown in AppendixA. 51 4.20 An x/y plane of the large tilt g0 configuration before (left and center) and after (right) GBS. The g0 is coherent with the right grain. Atoms that have an FCC environment are colored dark blue (nickel grains) or white (g0), and all other atoms are colored according to centrosymmetry. 52 xi 4.21 An x/y plane of the large square g0 configuration before (left and center) and after (right) GBS. The g0 is coherent with the right grain. Atoms that have an FCC environment are colored dark blue (nickel grains) or white (g0), and all other atoms are colored according to centrosymmetry. 52 4.22 An x/y plane of the large inline g0 configuration before (left and center) and after (right) GBS. The g0 is coherent with the right grain. Atoms that have an FCC environment are colored dark blue (nickel grains) or white (g0), and all other atoms are colored according to centrosymmetry. 53 4.23 The (left) stress vs.
Details
-
File Typepdf
-
Upload Time-
-
Content LanguagesEnglish
-
Upload UserAnonymous/Not logged-in
-
File Pages92 Page
-
File Size-