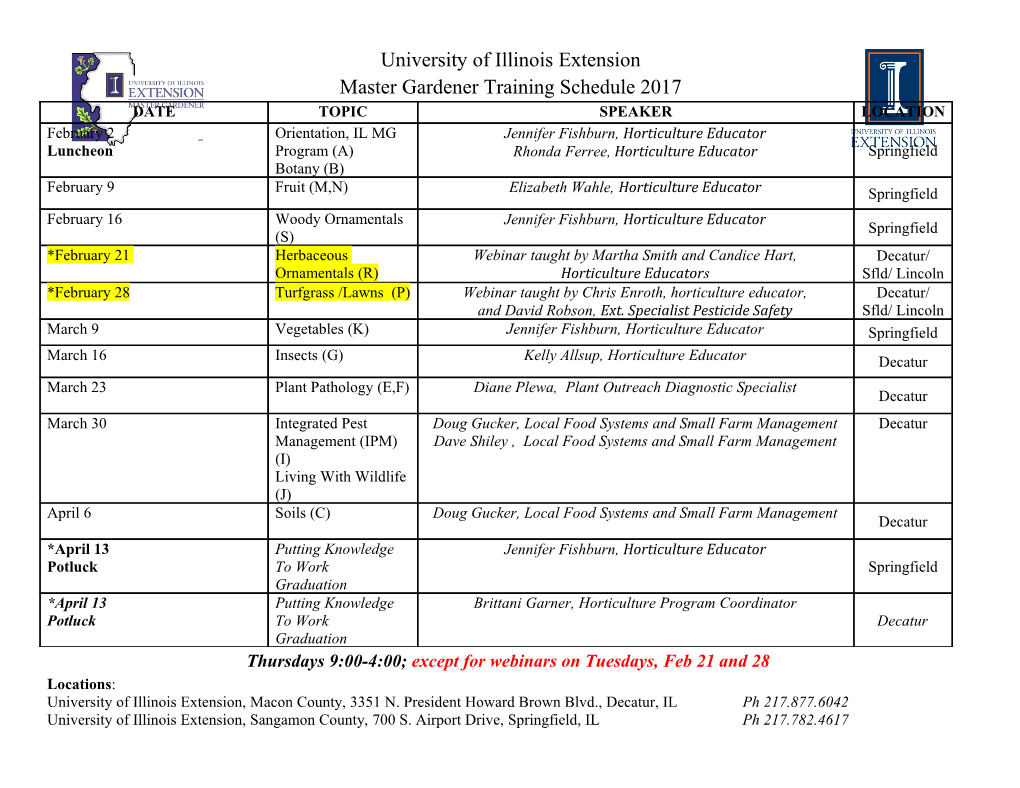
14 SEPARATION TECHNIQUES 14.1 Introduction The methods for separating, collecting, and detecting radionuclides are similar to ordinary analytical procedures and employ many of the chemical and physical principles that apply to their nonradioactive isotopes. However, some important aspects of the behavior of radionuclides are significantly different, resulting in challenges to the radiochemist to find a means for isolation of a pure sample for analysis (Friedlander et al., 1981). While separation techniques and principles may be found in standard textbooks, Chapter 14 addresses the basic chemical principles that apply to the analysis of radionuclides, with an emphasis on their unique behavior. It is not a comprehensive review of all techniques. This chapter provides: (1) a review of the important chemical principles underlying radiochemical separations, (2) a survey of the important separation methods used in radiochemistry with a discussion of their advantages and disadvantages, and (3) an examination of the particular features of radioanalytical chemistry that distinguish it from ordinary analytical chemistry. Extensive examples have been provided throughout the chapter to illustrate various principles, practices, and procedures in radiochemistry. Many were selected purposely as familiar illustrations from agency procedural manuals. Others were taken from the classical and recent radiochemical literature to provide a broad, general overview of the subject. This chapter integrates the concepts of classical chemistry with those topics unique to radio- nuclide analysis. The first eight sections of the chapter describe the bases for chemical separations involving oxidation-reduction, complex-ion formation, distillation/volatilization, solvent extraction, precipitation and coprecipitation, electrochemistry, and chromatography. Carriers and tracers, which are unique to radiochemistry, are described in Section 14.9 together with specific separation examples for each of the elements covered in this manual. Section 14.10 also provides an overview of the solution chemistry Contents and appropriate separation techniques for 17 elements. An attachment at the end of the chapter 14.1 Introduction .................... 14-1 describes the phenomenon of radioactive 14.2 Oxidation-Reduction Processes ..... 14-2 equilibrium, also unique to radioactive materials. 14.3 Complexation .................. 14-18 14.4 Solvent Extraction .............. 14-25 14.5 Volatilization and Distillation ..... 14-36 Because the radiochemist detects atoms by their 14.6 Electrodeposition ............... 14-41 radiation, the success or failure of a radiochemical 14.7 Chromatography ............... 14-44 procedure often depends on the ability to separate 14.8 Precipitation and Coprecipitation . 14-56 extremely small quantities of radionuclides (e.g., 14.9 Carriers and Tracers ............ 14-82 10!6 to 10!12 g) that might interfere with detection 14.10 Analysis of Specific Radionuclides . 14-97 14.11 References ................... 14-201 of the analyte. For example, isolation of trace 14.12 Selected Bibliography .......... 14-218 quantities of a radionuclide that will not precipitate Attachment 14A Radioactive Decay and on its own with a counter-ion requires judicious Equilibrium .................. 14-223 JULY 2004 14-1 MARLAP Separation Techniques selection of a carrier and careful technique to produce a coprecipitate containing the pure radionuclide, free of interfering ions. In detection procedures, the differences in the behavior of radionuclides provide unique oppor- tunities not available in the traditional analytical chemistry of nonradioactive elements. Radio- nuclides often can be detected by their unique radiation regardless of the chemical form of the element. There is also a time factor involved because of the short half-lives of some radionuc- lides. Traditional procedures involving long digestion or slow filtration cannot be used for short- lived radionuclides, thereby requiring that rapid separations be developed. Another distinction is the hazards associated with radioactive materials. At very high activity levels, chemical effects of the radiation, such as decomposition of solvents (through radiolysis) and heat effects (caused by interaction of decay particles with the solution), can affect the procedures. Equally important, even at lower activity levels, is the radiation dose that the radiochemist can receive unless protected by shielding, ventilation, time, or distance. Even at levels where the health concerns are minimal, special care needs to be taken to guard against laboratory and equipment contamination. Moreover, the radiochemist should be concerned about the type and quantity of the waste generated by the chemical procedures employed, because the costs and difficulties associated with the disposal of low-level and mixed radioactive waste continue to rise (see Chapter 17, Waste Management in a Radioanalytical Laboratory). The past 10 years have seen significant improvements to some of the classical techniques as well as the development of new methods of radiochemical analysis. Knowledge of these analytical developments, as well as maintenance of a working familiarity with developing techniques in the radiochemistry field will further enhance the waste reduction effort. 14.2 Oxidation-Reduction Processes 14.2.1 Introduction Oxidation and reduction (redox) processes play an important role in radioanalytical chemistry, particularly from the standpoint of the dissolution, separation, and detection of analytes, tracers, and carriers. Ion exchange, solvent extraction, and solid-phase extraction separation techniques, for example, are highly dependent upon the oxidation state of the analytes. Moreover, most radiochemical procedures involve the addition of a carrier or isotope tracer. There must be complete equilibration (isotopic exchange) between the added isotope(s) and all the analyte species present in order to achieve quantitative yields. The oxidation number of a radionuclide can affect its chemical stability in the presence of water, oxygen, and other natural substances in solution; reactivity with reagents used in the radioanalytical procedure; solubility in the presence of other ions and molecules; and behavior in the presence of carriers and tracers. The oxidation numbers of radionuclides in solution and their susceptibility to change, because of natural or induced redox processes, are critical, therefore, to the physical and chemical behavior of MARLAP 14-2 JULY 2004 Separation Techniques radionuclides during these analytical procedures. The differences in mass number of all radionuclides of an element are so small that they will exhibit the same chemical behavior during radiochemical analysis (i.e., no mass isotope effects). 14.2.2 Oxidation-Reduction Reactions An oxidation-reduction reaction (redox reaction) is a reaction in which electrons are redistributed among the atoms, molecules, or ions in solution. In some redox reactions, electrons are actually transferred from one reacting species to another. Oxidation under these conditions is defined as the loss of electron(s) by an atom or other chemical species, whereas reduction is the gain of electron(s). Two examples will illustrate this type of redox reaction: +6 !1 U + 3 F2 6 U + 6 F Pu+4 + Fe+2 6 Pu+3 + Fe+3 In the first reaction, uranium loses electrons, becoming a cation (oxidized), and fluorine gains an electron (reduced), becoming an anion. In the second reaction, the reactants are already ions, but the plutonium cation (Pu+4) gains an electron, becoming Pu+3 (reduced), and the ferrous ion (Fe+2) loses an electron, becoming Fe+3 (oxidized). In other redox reactions, electrons are not completely transferred from one reacting species to another; the electron density of one atom decreases while it increases at another atom. The change in electron density occurs as covalent bonds (in which electrons are shared between two atoms) are broken or made during a chemical reaction. In covalent bonds between two atoms of different elements, one atom is more electronegative than the other atom. Electronegativity is the ability of an atom to attract electrons in a covalent bond. One atom, therefore, attracts the shared pair of electrons more effectively, causing a difference in electron density about the atoms in the bond. An atom that ends up bonded to a more electronegative atom at the end of a chemical reaction loses net electron density. Conversely, an atom that ends up bonded to a less electro- negative atom gains net electron density. Electrons are not transferred completely to other atoms, and ions are not formed because the electrons are still shared between the atoms in the covalent bond. Oxidation, in this case, is defined as the loss of electron density, and reduction is defined as the gain of electron density. When carbon is oxidized to carbon dioxide by oxygen: C + O2 6 CO2 the electron density associated with the carbon atom decreases, and that of the oxygen atoms increases, because the electronegativity of oxygen is greater than the electronegativity of carbon. In this example, carbon is oxidized and oxygen is reduced. Another example from the chemistry of the preparation of gaseous uranium hexafluoride (UF6) illustrates this type of redox reaction: JULY 2004 14-3 MARLAP Separation Techniques 3 UF4 + 2 ClF3 6 3 UF6 + Cl2 Because the order of electronegativity of the atoms increases in the order U < Cl < F, the uranium atom in uranium tetrafluoride (UF4) is oxidized further
Details
-
File Typepdf
-
Upload Time-
-
Content LanguagesEnglish
-
Upload UserAnonymous/Not logged-in
-
File Pages232 Page
-
File Size-