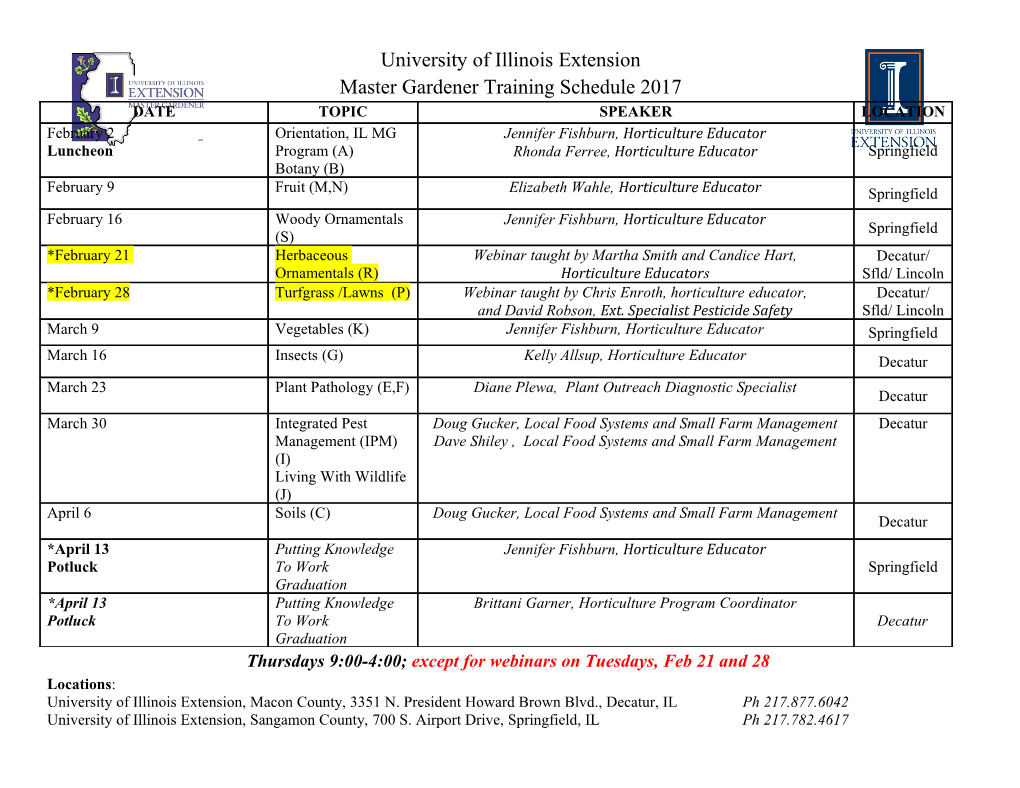
Acta Biomaterialia 13 (2015) 364–373 Contents lists available at ScienceDirect Acta Biomaterialia journal homepage: www.elsevier.com/locate/actabiomat Field effect transistors based on semiconductive microbially synthesized chalcogenide nanofibers ⇑ Ian R. McFarlane a, Julia R. Lazzari-Dean b, Mohamed Y. El-Naggar a,c, a Department of Physics and Astronomy, University of Southern California, 920 Bloom Walk, Seaver Science Center 215C, Los Angeles, CA 90089-0484, USA b Department of Chemistry, University of Southern California, Los Angeles, CA 90089-0484, USA c Molecular and Computational Biology Section, Department of Biological Sciences, University of Southern California, Los Angeles, CA 90089-0484, USA article info abstract Article history: Microbial redox activity offers a potentially transformative approach to the low-temperature synthesis of Received 27 June 2014 nanostructured inorganic materials. Diverse strains of the dissimilatory metal-reducing bacteria Received in revised form 3 October 2014 Shewanella are known to produce photoactive filamentous arsenic sulfide nanomaterials by reducing Accepted 4 November 2014 arsenate and thiosulfate in anaerobic culture conditions. Here we report in situ microscopic observations Available online 11 November 2014 and measure the thermally activated (79 kJ molÀ1) precipitation kinetics of high yield (504 mg per liter of culture, 82% of theoretical maximum) extracellular As2S3 nanofibers produced by Shewanella sp. strain Keywords: ANA-3, and demonstrate their potential in functional devices by constructing field effect transistors Shewanella (FETs) based on individual nanofibers. The use of strain ANA-3, which possesses both respiratory and Arsenic sulfide Nanostructures detoxification arsenic reductases, resulted in significantly faster nanofiber synthesis than other strains Field effect transistor previously tested, mutants of ANA-3 deficient in arsenic reduction, and when compared to abiotic arsenic 2 Biogenic materials sulfide precipitation from As(III) and S À. Detailed characterization by electron microscopy, energy-dis- persive X-ray spectroscopy, electron probe microanalysis and Tauc analysis of UV-vis spectrophotometry showed the biogenic precipitate to consist primarily of amorphous As2S3 nanofibers with an indirect optical band gap of 2.37 eV. X-ray diffraction also revealed the presence of crystalline As8S9-x minerals that, until recently, were thought to form only at higher temperatures and under hydrothermal condi- tions. The nanoscale FETs enabled a detailed characterization of the charge mobility (10À5 cm2 VÀ1 sÀ1) and gating behavior of the heterogeneously doped nanofibers. These studies indicate that the biotrans- formation of metalloids and chalcogens by bacteria enables fast, efficient, sustainable synthesis of technologically relevant chalcogenides for potential electronic and optoelectronic applications. Ó 2014 Acta Materialia Inc. Published by Elsevier Ltd. This is an open access article under the CC BY-NC- ND license (http://creativecommons.org/licenses/by-nc-nd/3.0/). 1. Introduction biominerals, especially carbonates and phosphates, the last decade has also witnessed additional interest in exploiting biological, The synthesis of nanomaterials by biological or biomimetic especially microbial, strategies for producing a wider range of syn- means in physiological conditions offers multiple advantages over thetic materials with technologically relevant mechanical, optical, traditional physical and chemical strategies that typically require electronic and magnetic functionalities [2–4]. Towards this goal, more extreme environments (temperature, pressure and pH). In recent reports [5–7] demonstrated the synthesis of extracellular addition to the promise of cheaper and greener synthesis pro- chalcogenide nanostructures with unique optoelectronic proper- cesses, the resulting biogenic materials can exhibit unique mor- ties using a bacterial process relying on anaerobic respiration and phologies and physical/chemical properties stemming from the detoxification activities to alter the oxidation states of the metal, tight control organisms exert over the composition, nucleation, metalloid and chalcogen precursors. crystallography and desired function of these materials [1]. While Chalcogenide compounds, resulting from the reaction of group significant attention has been dedicated to understanding the syn- VI elements (particularly S, Se and Te) with more electropositive thesis and structure–function relations of the most abundant elements (e.g. As, Sb, Si, Ge, Zn, Cd), represent an intriguing target for biogenic synthesis. Chalcogenides have been described as ‘‘cha- ⇑ Corresponding author at: Department of Physics and Astronomy, University of meleon’’ compounds because of their remarkable versatility: Southern California, 920 Bloom Walk, Seaver Science Center 215C, Los Angeles, CA depending on composition and synthesis techniques they may be 90089-0484, USA. Tel.: +1 (213) 740 2394; fax: +1 (213) 740 6653. crystalline, glassy, metallic, semiconductive or ionic conductors E-mail address: [email protected] (M.Y. El-Naggar). http://dx.doi.org/10.1016/j.actbio.2014.11.005 1742-7061/Ó 2014 Acta Materialia Inc. Published by Elsevier Ltd. This is an open access article under the CC BY-NC-ND license (http://creativecommons.org/licenses/by-nc-nd/3.0/). I.R. McFarlane et al. / Acta Biomaterialia 13 (2015) 364–373 365 [8]. This versatility leads to a wide range of tunable functionalities with an ATPase subunit, ArsA, to drive As(III) efflux using ATP in various components including sensors, waveguides, photonic hydrolysis. In contrast, the arr pathway has only been recently crystals [9] and photoactive devices [10–12]. Chalcogenide glasses described [18,21].ThearrAB operon of strain ANA-3 encodes a are already commercially important in write-once and rewritable large Mo-containing enzyme, ArrA, and an Fe–S protein, ArrB. optical storage disks as well as phase-change memory relying on Both ArrA and ArrB are required for respiratory reduction of amorphous–crystalline transformations [8]. Some members of this As(V) [18,19]. The use of strain ANA-3 resulted in significantly family (e.g. As2S3) are infrared-transparent (700 nm–11.5 lm) and more rapid precipitation of As–S nanofibers than previously are therefore candidate materials for applications in infrared reported under similar conditions. Furthermore, we demonstrate devices [9,13]. From an energy conversion standpoint, chalcoge- novel field-effect transistors (FETs) based on single biogenic nides have been intensively investigated as photovoltaic materials nanofibers, and study their charge mobility and switching behav- for solar cells [12]. In addition, AsxSy and AsxSey glasses have been ior as a function of backgating to identify the doping type and reported to exhibit an optomechanical effect [10,11] for direct majority charge carriers in these semiconductors. light-to-mechanical energy conversion when irradiated with polar- ized light matching the band gap energy. This phenomenon has 2. Materials and methods been exploited to generate mechanical strain, resulting in all-opti- cal (electricity-free) actuation of chalcogenide-coated cantilevers 2.1. Bacterial growth [10]. Further interest in unique and tunable size-dependent prop- erties has motivated the development of quasi-one-dimensional A list of the bacterial strains and mutants used in this study is (nanotube and nanowire) chalcogenides. The majority of these provided in Table 1. The inocula were grown aerobically in 20 ml efforts, however, focus on physical and chemical synthesis tech- of LB medium from a frozen (À80 °C) stock up to an optical density niques requiring templates, precursors, and hydro- or solvothermal at 600 nm (OD ) of 1.5 ± 0.15. These aerobic pre-cultures were methods, typically under relatively extreme conditions [14]. 600 inoculated at 0.1% (v/v) into anaerobic serum bottles each contain- More recently, Hur et al. reported a microbiological route for ing 80 mL HEPES-buffered (30 mM) medium consisting of: 20 mM synthesizing chalcogenide nanostructures by exploiting dissimila- sodium DL-lactate, as electron donor; 28 mM ammonium chloride; tory metal-reducing bacteria, specifically a number of Shewanella 1.34 mM potassium chloride; 4.35 mM sodium phosphate species, to produce an extracellular network of filamentous arsenic monobasic; 20 mM sodium hydroxide; 10 mM thiosulfate, as sulfide nanofibers/nanotubes in anaerobic culture conditions [5,6]. Na S O Á5H O; and 5 mM arsenate, as Na HAsO Á7H O. Vitamins, This process relies on the remarkable metabolic versatility of 2 2 3 2 2 4 2 amino acids and trace mineral stock solutions were used to Shewanella [15,16] to concomitantly reduce S O2À to S2À and 2 3 supplement the medium as described previously [22]. The medium As(V) to As(III). In this system, the thiosulfate S O2À serves as a ter- 2 3 was adjusted to an initial pH of 7.25, and anaerobic conditions were minal electron acceptor for respiration in lieu of O under anaero- 2 reached by purging with 100% N for in excess of 45 min. The bic conditions, resulting in S2À, while arsenate reduction is thought 2 anaerobic serum bottles, sealed with butyl stoppers and aluminum to occur via As reductases tied to either the respiration or detoxi- seals, were sterilized by autoclaving at 120 °C for 15 min. Arsenate, fication pathways of Shewanella. Rather surprisingly, it was thiosulfate and vitamins were added after autoclaving. All cultures reported that the resulting amorphous
Details
-
File Typepdf
-
Upload Time-
-
Content LanguagesEnglish
-
Upload UserAnonymous/Not logged-in
-
File Pages10 Page
-
File Size-