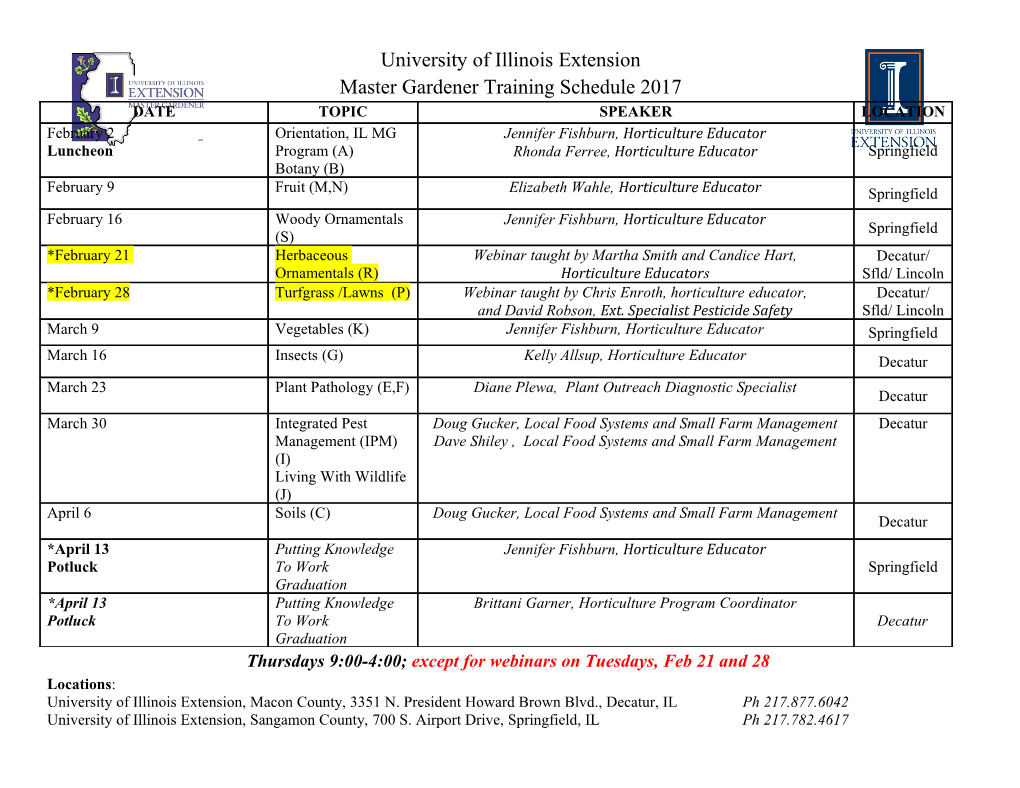
The Transfer Function The Transfer Function • A generalization of the frequency response • Taking the z-transforms of both sides we get function ∞ ∞ ∞ −n ⎛ ⎞ −n • The convolution sum description of an LTI Y(z) = ∑ y[n]z = ∑∑⎜ h[k]x[n − k]⎟ z discrete-time system with an impulse n=−∞ nk=−∞⎝ =−∞ ⎠ ∞ ∞ response h[n] is given by ⎛ −n ⎞ = ∑∑h[k]⎜ x[n − k]z ⎟ ∞ k=−∞ ⎝ n=−∞ ⎠ y[n] = ∑h[k]x[n − k] ∞ ⎛ ∞ ⎞ k=−∞ −(l+k) = ∑∑h[k]⎜ x[l]z ⎟ k=−∞ =−∞ 1 2 ⎝ l ⎠ Copyright © 2005, S. K. Mitra Copyright © 2005, S. K. Mitra The Transfer Function The Transfer Function ∞ ⎛ ∞ ⎞ • Hence, −l −k •Or,Y(z) = ∑∑h[k]⎜ x[l]z ⎟ z H (z) = Y(z)/ X (z) k=−∞ ⎝ =−∞ ⎠ l • The function H(z), which is the z-transform of X (z) the impulse response h[n] of the LTI system, ⎛ ∞ ⎞ −k is called the transfer function or the system • Therefore, Y(z) = ⎜ ∑h[k]z ⎟ X (z) ⎝ k=−∞ ⎠ function H(z) • The inverse z-transform of the transfer function H(z) yields the impulse response h[n] • Thus, Y(z) = H(z)X(z) 3 4 Copyright © 2005, S. K. Mitra Copyright © 2005, S. K. Mitra The Transfer Function The Transfer Function • Consider an LTI discrete-time system characterized by a difference equation • Or, equivalently as M M −k ∑ pk z N d y[n − k] = M p x[n − k] H(z) = z(N −M ) k=0 ∑k=0 k ∑k=0 k N N −k ∑k=0 dk z • Its transfer function is obtained by taking • An alternate form of the transfer function is the z-transform of both sides of the above given by equation M −1 M −k p0 ∏k=1(1−ξk z ) ∑k=0 pk z H(z) = ⋅ • Thus H(z) = d N −1 N −k 0 ∏k=1(1− λk z ) ∑k=0 dk z 5 6 Copyright © 2005, S. K. Mitra Copyright © 2005, S. K. Mitra 1 The Transfer Function The Transfer Function • Or, equivalently as • For a causal IIR digital filter, the impulse M response is a causal sequence p ∏ (z −ξk ) H(z) = 0 z(N −M ) k=1 N • The ROC of the causal transfer function d0 (z − λ ) ∏k=1 k M p0 (N −M ) ∏k=1(z −ξk ) • ξ1, ξ2,...,ξM are the finite zeros, and H(z) = z d N λ1, λ2,...,λN are the finite poles of H(z) 0 ∏k=1(z − λk ) •IfN > M, there are additional ( N − M ) zeros is thus exterior to a circle going through the at z = 0 pole furthest from the origin •IfN < M, there are additional ( M − N ) poles • Thus the ROC is given by z > maxλk k at z = 0 7 8 Copyright © 2005, S. K. Mitra Copyright © 2005, S. K. Mitra The Transfer Function The Transfer Function • The transfer function has M zeros on the •Example- Consider the M-point moving- unit circle at z = e j 2 π k / M , 0 ≤ k ≤ M −1 average FIR filter with an impulse response • There are M − 1 poles at z = 0 and a single ⎧1/ M , 0 ≤ n ≤ M −1 pole at z = 1 M = 8 h[n] = ⎨ ⎩ 0, otherwise • The pole at z = 1 1 • Its transfer function is then given by exactly cancels the 0.5 7 0 M −1 −M M zero at z = 1 1 1− z z −1 Imaginary-0.5 Part H (z) z−n = = = ∑ −1 M • The ROC is the entire M n=0 M (1− z ) M[z (z −1)] -1 z-plane except z = 0 -1 -0.5 0 0.5 1 Real Part 9 10 Copyright © 2005, S. K. Mitra Copyright © 2005, S. K. Mitra The Transfer Function The Transfer Function • Alternate forms: z2 −1.2z +1 •Example- A causal LTI IIR digital filter is H (z) = described by a constant coefficient z3 −1.3z2 +1.04z − 0.222 difference equation given by (z − 0.6 + j0.8)(z − 0.6 − j0.8) = y[n] = x[n −1]−1.2 x[n − 2]+ x[n − 3]+1.3 y[n −1] (z − 0.3)(z − 0.5 + j0.7)(z − 0.5 − j0.7) 1 −1.04 y[n − 2]+ 0.222 y[n − 3] •Note:Poles farthest from 0.5 • Its transfer function is therefore given by z = 0 have a magnitude 0 z−1 −1.2z−2 + z−3 Imaginary-0.5 Part H (z) = 0.74 −1 −2 −3 -1 1−1.3z +1.04z − 0.222z -1 -0.5 0 0.5 1 11 12 • ROC: z > 0 . 74 Real Part Copyright © 2005, S. K. Mitra Copyright © 2005, S. K. Mitra 2 Frequency Response from Frequency Response from Transfer Function Transfer Function • If the ROC of the transfer function H(z) • For a stable rational transfer function in the includes the unit circle, then the frequency form M H (e jω) p ∏ (z −ξk ) response of the LTI digital filter can H(z) = 0 z(N −M ) k=1 be obtained simply as follows: d N 0 ∏k=1(z − λk ) H (e jω) = H (z) z=e jω the factored form of the frequency response • For a real coefficient transfer function H(z) is given by it can be shown that M jω p ∏ (e − ξk ) jω 2 jω jω H (e jω) = 0 e jω(N −M ) k=1 H (e ) = H (e )H *(e ) d N jω jω − jω −1 0 ∏k=1(e − λk ) = H (e )H (e ) = H (z)H (z ) jω 13 z=e 14 Copyright © 2005, S. K. Mitra Copyright © 2005, S. K. Mitra Frequency Response from Frequency Response from Transfer Function Transfer Function • It is convenient to visualize the contributions which reduces to M jω of the zero factor (z − ξ )and the pole factor p ∏ e − ξk k H (e jω) = 0 k=1 (z − λ ) from the factored form of the N jω k d0 e − λ frequency response ∏k=1 k • The phase response for a rational transfer • The magnitude function is given by function is of the form M jω p ∏ e − ξk jω H (e jω) = 0 e jω(N −M ) k=1 arg H (e ) = arg( p0 / d0) + ω(N − M ) d N jω M N 0 ∏k=1 e − λk jω jω + ∑arg(e − ξk ) − ∑arg(e − λk ) 15 16 k=1 k=1 Copyright © 2005, S. K. Mitra Copyright © 2005, S. K. Mitra Frequency Response from Geometric Interpretation of Transfer Function Frequency Response Computation • The magnitude-squared function of a real- • The factored form of the frequency coefficient transfer function can be response M jω computed using p ∏ (e − ξk ) H (e jω) = 0 e jω(N −M ) k=1 N 2 M d jω jω − jω * 0 ∏k=1(e − λk ) jω 2 p0 ∏k=1(e − ξk )(e − ξk ) H (e ) = is convenient to develop a geometric d N (e jω − λ )(e− jω − λ* ) 0 ∏k=1 k k interpretation of the frequency response computation from the pole-zero plot as ω varies from 0 to 2π on the unit circle 17 18 Copyright © 2005, S. K. Mitra Copyright © 2005, S. K. Mitra 3 Geometric Interpretation of Geometric Interpretation of Frequency Response Computation Frequency Response Computation • The geometric interpretation can be used to • As shown below in the z-plane the factor obtain a sketch of the response as a function (e jω − ρe jφ) represents a vector starting at of the frequency the point z = ρ e jφ and ending on the unit jω • A typical factor in the factored form of the circle at z = e frequency response is given by (e jω − ρe jφ) where ρ e jφ is a zero if it is zero factor or is a pole if it is a pole factor 19 20 Copyright © 2005, S. K. Mitra Copyright © 2005, S. K. Mitra Geometric Interpretation of Geometric Interpretation of Frequency Response Computation Frequency Response Computation •As ω is varied from 0 to 2π, the tip of the • As indicated by M jω vector moves counterclockise from the p ∏ e − ξk H (e jω) = 0 k=1 point z = 1 tracing the unit circle and back N jω d0 ∏ e − λk to the point z = 1 k=1 the magnitude response | H ( e j ω ) | at a specific value of ω is given by the product of the magnitudes of all zero vectors divided by the product of the magnitudes of all pole vectors 21 22 Copyright © 2005, S. K. Mitra Copyright © 2005, S. K. Mitra Geometric Interpretation of Geometric Interpretation of Frequency Response Computation Frequency Response Computation • Likewise, from jω arg H (e ) = arg( p0 / d0) + ω(N − M ) • Thus, an approximate plot of the magnitude + ∑M arg(e jω − ξ ) − ∑N arg(e jω − λ ) and phase responses of the transfer function k=1 k k=1 k of an LTI digital filter can be developed by we observe that the phase response examining the pole and zero locations at a specific value of ω is obtained by • Now, a zero (pole) vector has the smallest adding the phase of the term p / d and the 0 0 magnitude when ω = φ linear-phase term ω ( N − M ) to the sum of the angles of the zero vectors minus the angles of the pole vectors 23 24 Copyright © 2005, S. K. Mitra Copyright © 2005, S. K. Mitra 4 Geometric Interpretation of Stability Condition in Terms of Frequency Response Computation Frequency Response Computation the Pole Locations • To highly attenuate signal components in a • A causal LTI digital filter is BIBO stable if specified frequency range, we need to place and only if its impulse response h[n] is zeros very close to or on the unit circle in absolutely summable, i.e., this range ∞ • Likewise, to highly emphasize signal S = ∑ h[n] < ∞ components in a specified frequency range, n=−∞ we need to place poles very close to or on • We now develop a stability condition in the unit circle in this range terms of the pole locations of the transfer function H(z) 25 26 Copyright © 2005, S.
Details
-
File Typepdf
-
Upload Time-
-
Content LanguagesEnglish
-
Upload UserAnonymous/Not logged-in
-
File Pages7 Page
-
File Size-