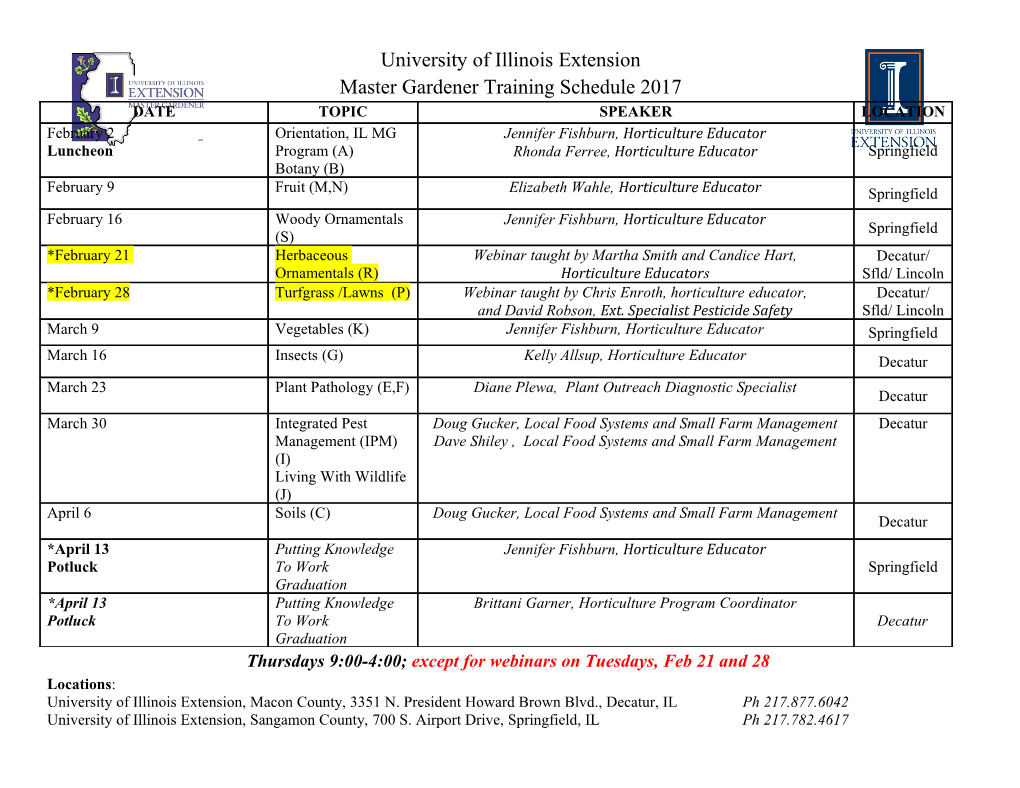
MICROSCOPY RESEARCH AND TECHNIQUE 47:172–181 (1999) An Evaluation of Two-Photon Excitation Versus Confocal and Digital Deconvolution Fluorescence Microscopy Imaging in Xenopus Morphogenesis AMMASI PERIASAMY,1,2* PAUL SKOGLUND,2 COLTEN NOAKES,1,2 AND RAYMOND KELLER2 1W.M. Keck Center for Cellular Imaging, Gilmer Hall, University of Virginia, Charlottesville, Virginia 22903 2Department of Biology, Gilmer Hall, University of Virginia, Charlottesville, Virginia 22903 KEY WORDS two-photon excitation; confocal; digital deconvolution; point spread function; fluorescence microscopy; deep tissue imaging; green fluorescent protein; xenopus morphogenesis; embryos ABSTRACT The ability to visualize cell motility occurring deep in the context of opaque tissues will allow many currently intractable issues in developmental biology and organogenesis to be addressed. In this study, we compare two-photon excitation with laser scanning confocal and conventional digital deconvolution fluorescence microscopy, using the same optical configuration, for their ability to resolve cell shape deep in Xenopus gastrula and neurula tissues. The two-photon microscope offers better depth penetration and less autofluorescence compared to confocal and conventional deconvolution imaging. Both two-photon excitation and confocal microscopy also provide improved rejection of ‘‘out-of-focus’’ noise and better lateral and axial resolution than conventional digital deconvolution microscopy. Deep Xenopus cells are best resolved by applying the digital deconvolution method on the two-photon images. We have also found that the two-photon has better depth penetration without any degradation in the image quality of interior sections compared to the other two techniques. Also, we have demonstrated that the quality of the image changes at different depths for various excitation powers. Microsc. Res. Tech. 47:172–181, 1999. 1999 Wiley-Liss, Inc. INTRODUCTION reaching a single detector, the photomultiplier tube Fluorescence microscopy has become one of the com- (PMT). Because of the pinhole aperture, the entire monly used techniques to visualize structural informa- fluorescence signal collected by the objective lens is not used (Pawley, 1995; Shotton, 1989; Wilson and Shep- tion of living specimens. Furthermore, the technology pard, 1984). In order to increase the signal, increased development and new fluorescent probes such as green excitation laser intensity is used, but this will often fluorescent proteins have revolutionized light micros- introduce photobleaching and photodamage. Unlike the copy imaging in living biological samples (Bright and confocal configuration, digital deconvolution micros- Taylor, 1986; Chalfie et al., 1994; Inoue´ and Spring, copy uses the entire fluorescence signal collected by the 1997; Periasamy and Herman, 1994; Sullivan and Kay, objective lens, without the use of pinhole or UV optics, 1999; Tsien, 1989). The wide-field digital deconvolution to deliver the emitted light to two-dimensional high- and the laser scanning confocal helped biologists to sensitivity and linear CCD detectors. The ‘‘out-of-focus’’ improve the image quality of the cells from fixed and flare introduced into the image at different optical live specimens. But there was always a struggle in deep sections is reversed by computer deconvolution through tissue imaging and handling the UV fluorophore for the use of a point spread function (PSF) of the imaging cellular imaging, which was solved after the invention system (Agard et al., 1989). of two-photon excitation microscopy system (Denk et In contrast, the two-photon excitation has a potential al., 1990). There are comparisons between confocal and advantage over conventional widefield digital deconvo- two-photon excitation techniques (Hell et al., 1994; lution (DDM) or laser scanning confocal microscopy Potter, 1996) but to our knowledge such a comparison (LSCM) because of its intrinsic three-dimensional reso- for three systems to visualize the live thick biological lution and the absence of background fluorescence. In specimen is not available. two-photon excitation imaging, the photobleaching and A major problem in visualizing the structures of thick autofluorescence are considerably reduced since the tissue has been the contribution of a signal from above infrared pulsed laser light illumination occurs only at and below the plane of focus, which produces a back- ground glow and results in degradation of the image. To Contract grant sponsor: National Science Foundation; Contract grant number: remedy these problems and produce more reliable DBI-9604709; Contract grant sponsor: National Institutes of Health; Contract grant numbers: S10-RR13714, HD-36426; Contract grant sponsor: W.M. Keck three-dimensional data, the most widely used tech- Foundation; Contract grant sponsor: Academic Enhancement Program of the niques are digital deconvolution and confocal micros- University of Virginia. copy (Chen et al., 1995; Diaspro et al., 1996; Shaw, *Correspondence to: Dr. Ammasi Periasamy, W.M. Keck Center for Cellular Imag- ing, Gilmer Hall, Department of Biology, University of Virginia, Charlottesville, VA 1993; White et al., 1987). A confocal microscope uses a 22903. E-mail: [email protected] pinhole aperture to restrict the ‘‘out-of-focus’’ flare Received 30 July 1999; accepted in revised form 2 August 1999 1999 WILEY-LISS, INC. TPE COMPARED WITH LSCM AND DDM 173 the focal plane (Denk et al., 1990; Goppert-Mayer, 1931; RNA and Injections. Capped in vitro transcribed Hell et al., 1996; Konig et al., 1996; Lakowicz, 1997; RNA was prepared using the mMessage mMachine kit Periasamy, 1999; Piston et al., 1994; Potter et al., 1996; (Ambion). Plasmid encoding GAP-43-GFP was linear- So et al., 1995; Wokosin et al., 1996). In DDM and ized with Not I and transcribed with SP6 (Kim et al., LSCM, however, one-photon UV or visible light illumi- 1998). This fusion protein, produced in the lab of Paul nates the specimen throughout the whole field of view Garrity, combines a palymitoylation signal from GAP-43 and a considerable amount of photobleaching occurs with Green Fluorescent Protein (GFP) (Chalfie et al., above and below the focal plane. Two-photon excitation 1994), localizing GFP to the cell membrane. Embryos microscopy (TPEM) is an essential imaging system for were injected with 5–20 pg RNA in 3–4 separate thick tissue (deeper) cellular imaging and UV absorp- injections in the animal half of the embryo for animal tion fluorophores. caps and in various locations for intact embryo experi- Amphibian systems have been used to study the ments, including vegetal injections. Injections were by process of generating a vertebrate body axis for most of standard methods (Kintner, 1988). Briefly, needles this century. This process entwines sequential cascades pulled (Pul-1, WP Instruments Inc.) from 0.75-mm I.D. of inductive cell fate decisions with morphogenesis glass capillaries (Harvard Apparatus) are mounted on a (Spemann, 1938). The patterned cellular behaviors pressure injection system (Medical Systems Corp.) and driving morphogenesis at gastrulation in the frog Xeno- in vitro synthesized RNA is front loaded into the needle. pus laevis are thought to be identified (Domingo and Drop sizes are calibrated by means of a normalized Keller, 1995; Shih and Keller, 1992a,b), and currently reticule, and embryos are injected in 1 ϫ MBS, 5% represent the highest resolution cellular description of Ficoll, and transferred to 1/3 ϫ MBS after a minimum morphogenesis of early development in any vertebrate. of 2 hours. MBS is 88 mM NaCl, 0.82 mM MgSO4, 2.4 However, the cells comprising Xenopus embryos are not mM NaHCO3, 1.0 mM KCl, 0.33 mM Ca(N)3)2, 0.41 mM transparent unless fixed and cleared so that it allows CaCl2, 10 mM HepespH7.4. only a snapshot image of cells, which were undergoing the dynamic process of morphogenesis. For this reason, dynamic descriptions of cell behaviors based on stan- Instrumentation dard conventional microscopy in living frog tissue under- A schematic diagram of the DDM and LSCM system going morphogenesis reflects what is occurring on the is shown in Figure 1. The system consists of a Nikon surface of the embryo or explant, rather than a full PCM200 confocal microscope based on a galvanometer three-dimensional image including the baso-lateral as- point-scanning scan head was coupled to a side port of pects of surface cell behavior, or cells in deeper tissue the Nikon TE 200 inverted microscope equipped with layers. A reliable technique for visualizing the three- epi-fluorescence and transmitted illumination optics dimensional motility of living Xenopus cells in whole (Nikon Inc., Melville, NY). The epi-fluorescent light embryos or explants undergoing morphogenesis would source was a 100 W Hg arc lamp and a halogen lamp for not only allow confirmation of the spatially and tempo- transillumination with a long working distance con- rally patterned behavior thought to drive gastrulation, denser (0.52). A Plan Fluor 20X, NA 0.78 multiimmer- but would also significantly facilitate studies designed sion (water and oil) objective lens was used for the to elucidate the molecular basis for these behaviors. image acquisition both for the DDM and LSCM image A GFP-GAP-43 fusion protein is expressed in develop- acquisition mode. The Ludl (Ludl Electronic Products ing Xenopus embryos by means of RNA injection of the Ltd., Hawthrone, NY) neutral density (ND), excitation corresponding in vitro transcribed mRNA. This fusion and emission filter wheels were interfaced to the per- protein localizes the GFP fluorophore to cell mem- sonal computer through a controller (as shown in Fig. 1) branes by means of a palmitoylation signal, revealing and were driven by the C-Imaging (Compix, Inc., cell outlines under fluorescence microscopy to provide a Cranberry Township, PA) software. This software oper- convenient marker of cell shape. In this paper, we ates both the PCM2000 and DDM data acquisition evaluate three methods for visualizing living cell shapes system. The DDM and LSCM use an arc lamp and deep in tissues: two-photon, confocal, and deconvolu- lasers (argon-ion, 488 nm; HeNe, 543 nm) as light tion microscopy. The usefulness of applying these imag- sources, respectively.
Details
-
File Typepdf
-
Upload Time-
-
Content LanguagesEnglish
-
Upload UserAnonymous/Not logged-in
-
File Pages10 Page
-
File Size-