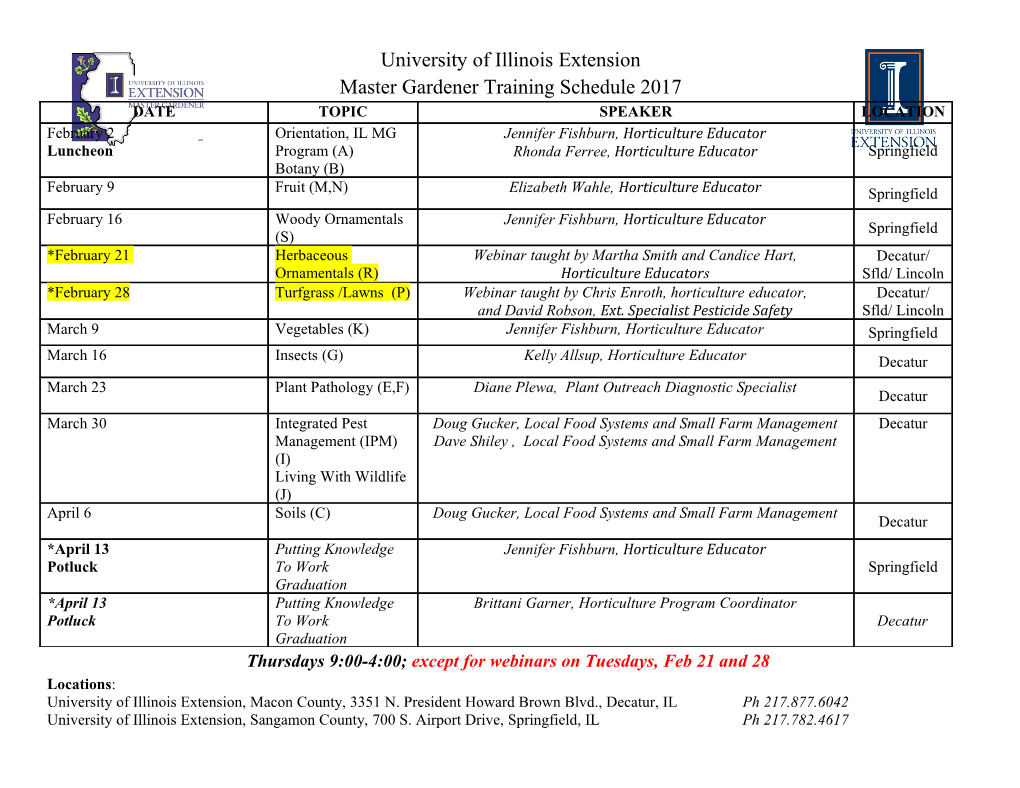
REVIEWS RECOMBINEERING: A POWERFUL NEW TOOL FOR MOUSE FUNCTIONAL GENOMICS Neal G. Copeland*, Nancy A. Jenkins* and Donald L. Court‡ Highly efficient phage-based Escherichia coli homologous recombination systems have recently been developed that enable genomic DNA in bacterial artificial chromosomes to be modified and subcloned, without the need for restriction enzymes or DNA ligases. This new form of chromosome engineering, termed recombinogenic engineering or recombineering, is efficient and greatly decreases the time it takes to create transgenic mouse models by traditional means. Recombineering also facilitates many kinds of genomic experiment that have otherwise been difficult to carry out, and should enhance functional genomic studies by providing better mouse models and a more refined genetic analysis of the mouse genome. MOUSE GENOMIC TECHNOLOGIES PHAGE-BASED RECOMBINATION During the next few years, the sequence of the human A limitation of most of these technologies is that Bacteria, such as Escherichia coli, genome will be completed and annotated. The next chal- they require the production of complicated targeting encode their own homologous lenge will be to determine how each of these genes func- and selection constructs. The ability to generate these recombination systems.Viruses tions alone and with other genes in the genome, to constructs is often limited by the availability of or phages that inhabit bacteria also often carry their own understand the developmental programme of a human. appropriate and unique restriction-enzyme cleavage recombination functions, which Given that there are many genes that need to be charac- sites in both cloning vectors and genomic DNA can work with, or independently terized and the fact that a lot of them will not be related to (BOX 1). Other types of functional genomics experi- of, the bacterial recombination genes with known function, this will be a daunting task. ment also require the manipulation of large segments functions. Much of our understanding of these genes will therefore of DNA, which is difficult to do using standard have to come from studies of model organisms. recombinant DNA techniques, because many cloning The mouse is an ideal model organism for these vectors do not have sufficient capacity or do not tend types of study. Not only are the mouse and human to tolerate large inserts. The development of PHAGE- genomes very similar, but also transgenic and knockout BASED HOMOLOGOUS RECOMBINATION systems in the past technologies, developed during the past two decades, three years5–8 has greatly simplified the generation of allow gene function studies in the mouse that are not transgenic and knockout constructs, making it possi- possible in most other organisms. Null mutations, as ble to engineer large segments of genomic DNA, such *Mouse Cancer Genetic well as subtle missense or gain-of-function mutations, as those carried on BACTERIAL ARTIFICIAL CHROMOSOMES ‡ Program, and Gene can be introduced into virtually any gene in the mouse (BACs) or P1 artificial chromosomes (PACs), that Regulation and Chromosome Biology germ line using homologous-recombination-based, replicate at low-copy number in Escherichia coli. Laboratory, Center for gene-targeting technology. In addition, the conditional Using phage recombination to carry out genetic engi- Cancer Research, National inactivation of gene expression in vivo can be used to neering has been called recombinogenic engineering9 Cancer Institute at inactivate a gene in only a subset of cells or at well- or recombineering. Recombineering offers exciting Frederick, Frederick, 1–4 Maryland 21702, USA. defined stages of development (for more on this new opportunities for creating mouse models of Correspondence to N.G.C. topic, see the accompanying review by Mark human disease and for gene therapy. Here, we con- e-mail: [email protected] Lewandoski on p743 of this issue). trast and compare these new phage systems with NATURE REVIEWS | GENETICS VOLUME 2 | OCTOBER 2001 | 769 © 2001 Macmillan Magazines Ltd REVIEWS Box 1 | Classical recombinant DNA technology versus recombineering Traditional genetic engineering technology, which uses restriction a Genetic engineering b Recombineering enzymes and DNA ligase to cut and rejoin DNA fragments (see Cassette PCR-amplified cassette figure part a), breaks down when cloning vehicles and the target site contain hundreds of kilobases of DNA. This is because even 5' 5' Restriction rare restriction-enzyme sites occur frequently on large DNA + molecules, such as bacterial artificial chromosomes (BACs) and Target in BAC the bacterial chromosome, making the finding of unique sites almost impossible. Furthermore, the in vitro manipulation of large Multi-copy linear DNAs of this length is extremely difficult; once BAC cloning plasmid drugR technology became available in Escherichia coli, modifying the BAC clones became the primary problem. Problems associated with the traditional approach have been overcome by developing ori recombineering technology, which uses homologous Ligation, recombination functions that are encoded by phages (see figure transformation Transformation Plasmid/phage- part b). The crucial advance in the recombineering methodology is mediated homologous the use of phage recombination functions that generate recombination recombinant molecules using homologies of 50 bp (or less); Recombinant cassette in BAC homologous regions for recombination usually need to be ~500 bp Cloned R cassette in length, whereas in recombineering approaches, they need be drug only 40–50 bp in length. (ori, origin of replication.) ori Genetic engineering steps to generate a BAC recombinant include: + • Cleaving a cassette DNA with a restriction enzyme. Target gene in BAC • Cleaving a target DNA on a plasmid with a restriction enzyme. • Ligating the cassette to the plasmid. • Transforming the plasmid into competent bacterial cells. • Selecting drug-resistant (drugR) clones. • Isolating the transformant and verifying the cloned cassette. • Transforming the cloned cassette into a BAC-containing bacterial strain to introduce the cloned cassette into the BAC by Classical homologous recombination steps homologous recombination. Regions of homology Target gene in BAC Recombinant cassette in BAC Recombineering steps to generate a BAC recombinant include: Restriction site • Amplifying a cassette by PCR with flanking regions of Plasmid DNA homology. BAC DNA • Introducing phage recombination functions into a BAC- PCR primers containing bacterial strain, or introducing a BAC into a strain Restriction enzyme that carries recombination functions. • Transforming the cassette into cells that contain a BAC and recombination functions. • Generating a recombinant in vivo. • Detecting a recombinant by selection, counterselection or by direct screening (colony hybridization). earlier, more classical bacterial recombination sys- tion pathway is very efficient10, making yeast a useful tems that have been used for engineering genomic organism for creating recombinant DNA molecules by DNA on BACs or PACs. homologous recombination. These recombination pathways recombine transformed, linear, double- Chromosome engineering in yeast stranded DNA (dsDNA) with homologous sites in the BACTERIAL ARTIFICIAL CHROMOSOME E. coli has traditionally been the main organism in yeast genome. Moreover, recombination occurs profi- (BAC). A cloning vector derived which to carry out genetic engineering for genetic stud- ciently with even short stretches of homologous from a single-copy F-plasmid of ies of bacteria, and also for constructing recombinant sequence, thereby allowing recombinant DNA to be Escherichia coli. It carries the F DNA molecules for use in other organisms. Most E. coli generated in vivo without the use of restriction replication and partitioning 11–13 systems that ensure low-copy cloning methods use restriction enzymes to cleave enzymes and DNA ligases . number and faithful DNA, and DNA ligases to join DNA fragments. These Homologous recombination has proved to be segregation of plasmid DNA to cleavage and joining reactions are the basis for creating extremely useful for analysing the function of genes in daughter cells. Large genomic recombinant molecules, and for cloning DNA into the yeast genome. In 1993, Agnès Baudin and fragments can be cloned into phage and PLASMID vectors for additional modification colleagues13 showed that it is possible to delete a yeast F-type plasmids, making them useful for constructing and amplification. In Saccharomyces cerevisiae, the gene and replace it with a yeast selectable marker, such genomic libraries. DNA double-stranded-break and -repair recombina- as HIS3, using homologous recombination. In these 770 | OCTOBER 2001 | VOLUME 2 www.nature.com/reviews/genetics © 2001 Macmillan Magazines Ltd REVIEWS 24,26–28 Box 2 | YACs versus BACs and PACs for recombineering activity to recBC mutants . These exonuclease- deficient strains provided one of the first in vivo cloning Yeast artificial chromosomes (YACs) were developed for cloning large fragments of systems for E. coli and have been used for several applica- genomic DNA into yeast, whereas bacterial artificial chromosomes (BACs) and P1 tions, including recombining linear dsDNA with circular artificial chromosomes (PACs) were developed for cloning large genomic fragments chromosomal DNA29, introducing drug-selectable into Escherichia coli. Each vector type has its own inherent advantages and markers into the E. coli chromosome, and for mutage-
Details
-
File Typepdf
-
Upload Time-
-
Content LanguagesEnglish
-
Upload UserAnonymous/Not logged-in
-
File Pages11 Page
-
File Size-