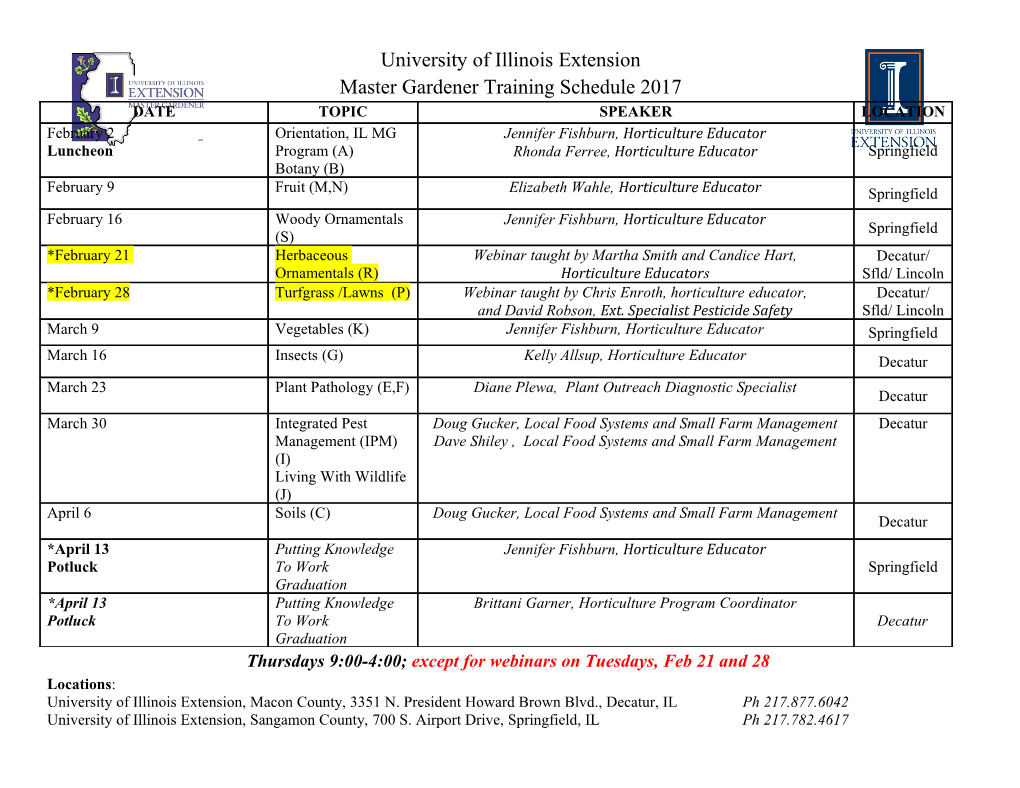
RF and Crosstalk Analysis of Copper and MLGNR Interconnects Using Different Repeaters in Sub-10 nm Regime Manjit Kaur Punjab Engineering College: PEC University of Technology Sanjeev Kumar Punjab Engineering College: PEC University of Technology Balwinder Raj National Institute of Technical Teachers' Training and Research Chandigarh Neena Gupta Punjab Engineering College: PEC University of Technology Arun Kumar Singh ( [email protected] ) Punjab Engineering College: PEC University of Technology https://orcid.org/0000-0003-0853-398X Research Article Keywords: Equivalent Single Conductor (ESC). Energy-delay-product (EDP). Far-End Crosstalk (FEXT). In- Phase Crosstalk (IPXT). Multilayer graphene nanoribbon (MLGNR). Near-End Crosstalk (NEXT). Out-of- Phase Crosstalk (OPXT) Posted Date: March 2nd, 2021 DOI: https://doi.org/10.21203/rs.3.rs-236432/v1 License: This work is licensed under a Creative Commons Attribution 4.0 International License. Read Full License RF and Crosstalk Analysis of Copper and MLGNR Interconnects Using Different Repeaters in Sub-10 nm Regime Manjit Kaur1, Sanjeev Kumar2, Balwinder Raj3, Neena Gupta1, and Arun K. Singh1* 1 Department of Electronics and Communication Engineering, Punjab Engineering College (Deemed to be University), Chandigarh, India 2 Department of Applied Sciences, Punjab Engineering College (Deemed to be University), Chandigarh, India 3 Department of Electronics and Communication Engineering, National Institute of Technical Teachers Training & Research, Sector 26, Chandigarh, India 1* Corresponding author e-mail: [email protected] Abstract This paper presents RF and crosstalk analysis of Copper (Cu) and multi-layer Graphene nanoribbon (MLGNR) based interconnects using multi-gate (FinFET) and virtual-source carbon nanotube field effect transistor (CNFET) based repeater insertions in sub-10 nm regime. The SPICE based analysis utilizes an accurate π-type equivalent single conductor (ESC) model for mutually coupled interconnects at 7 nm technology node. The transfer function and 3-dB bandwidth results of lithium-doped MLGNRs offer many fold improved RF performance than Cu. The out-of-phase crosstalk induced (OPXT) delay results with FinFET repeaters demonstrate 27.54 and 67.6 % reductions for pristine and lithium-doped MLGNRs as compared to Cu, whereas CNFET repeaters demonstrate 20.48 and 81.88 % reductions at interconnect length of 1000 µm. The peak far-end crosstalk (FEXT) noise voltage results demonstrate 86.03 and 62.5 % using FinFET repeaters and 88.14 and 69.9 % reductions using CNFET repeaters for pristine and Li-doped MLGNRs than Cu at 1000 µm length. Further, the energy-delay-product (EDP) results demonstrate 59.7 and 97 % reductions using FinFET repeaters for pristine and Li-doped MLGNRs than Cu at length of 1000 µm. The EDP results using CNFET repeaters exhibit 34 % degradations for pristine-MLGNRs than Cu while Li-MLGNR exhibit 98.61% reductions than Cu at length of 1000 µm. Keywords Equivalent Single Conductor (ESC). Energy-delay-product (EDP). Far-End Crosstalk (FEXT). In-Phase Crosstalk (IPXT). Multilayer graphene nanoribbon (MLGNR). Near-End Crosstalk (NEXT). Out-of-Phase Crosstalk (OPXT) 1. Introduction The continual feature size shrinking in modern integrated circuits (ICs) has paved the way for performance enhancements. However, at nanoscale technology nodes there starts a greater disparity in scaling factors of transistors and interconnects. This resulted in making interconnects a limiting factors in overall system performance. Moreover, the performance of CMOS transistors have started degrading significantly at nanoscale nodes. So, research community is coming up with new interconnect materials and alternatives of CMOS transistors for future ICs. The international technology roadmaps for semiconductor (ITRS) has forecasts that existing widely used interconnect material Cu is going to cross its critical current density value very soon [1]. Emerging interconnect materials having novel properties to overcome limitations of Cu at nanoscale. Carbon based GNR nanomaterials are becoming top choice of researchers to explore them for interconnect applications due to their scalability to nanoscale and novel properties such as two-dimensional current flow, ballistic transport, very high current handling capacity, larger mean free path, greater thermal stability, and improved mechanical strength [1-3]. Further, greater momentum relaxation time and the planar structure facilitates smooth control of their chiral vectors during fabrication process. This results in easy fabrication either as semiconducting or metallic as per requirement. Hence, GNRs are evolving as potential replacement of Cu for chip interconnect applications. Basically, a GNR comprise of a strip of Graphene sheet with width generally less than 50 nm. They are further categorized into armchair (ac) and zig-zag (zz) depending on profile of their edges. Normally, many Graphene layers are stacked to enhance conductivity and it is known as multi-layer GNRs or MLGNRs. The signal integrity degradation due to higher packing density, dielectric imperfections, EMI/EMC issues, and skin effect etc. worsen at higher frequencies and could not be omitted in modern integrated circuits (ICs) [1-3]. So, the necessity of radio frequency and crosstalk analysis in performance characterization of chip interconnects has arisen in modern ICs. With increasing switching rate of signalling, inductance has started playing dominant role in nanoscale ICs. The global level interconnects are generally wider wires having higher conductivity as compared to local/intermediate level. So, they demonstrate larger inductive related activities. This necessitates inclusion of inductance as important parameter in modelling of emerging as well Cu interconnects at nanoscale technology nodes. It has been demonstrated that exclusion of inductance in interconnect models may result in errors up to 35% for propagation delay calculations [4]. The scaling down of feature size below 22 nm raised many issues at process and device level which has led to search for new device structures [1, 3]. Multi-gate transistors generally called Fin-FETs due to their Fin type shape are just structural improvement of traditional CMOS. They demonstrate better gate control over channel current with minimal presence of short channel effects (SCEs) as reported in [5-7]. CNFET has attracted attention of many researchers due to use of CNTs as channel material and their novel properties [8]. A CNFET compact model supporting multiple chiralties, diameters, and semiconducting/metallic CNT based channels has been introduced by Deng et al.[9]. This model includes optical/ acoustical phonon scattering, charge screening, and quantum confinement etc. Deng and Wong then demonstrated standard HSPICE CNFET model considering experimental device non-linearities [10]. Lee et al. introduced a virtual-source based semi-empirical compact CNFET model which appropriately considers effects of geometrical scaling, tunnelling currents, and parasitic resistance/capacitance etc. [11-12]. The virtual–source CNFET model (VSCNFET) extends CNFET technology to sub-10-nm technology nodes [13]. The RF analysis of MLGNRs is discussed by only few researchers [14-17]. The skin-depth and surface impedance based high frequency performance of pristine and intercalated MLGNRs is studied in [14-15]. However, the validation of RF behaviour at circuit level is missing. The Bode plots based relative stability of Cu, side-contact (SC) and top-contact (TC) MLGNRs is investigated in [16] for only local/intermediate level pristine MLGNR omitting the global level interconnects. Further, A research article compared MLGNRs and MWCNTs using driver-interconnect-load (DIL) system in terms absolute transfer function and bandwidth [17]. To improve the performance of nowadays ICs, repeaters are invariably inserted into longer resistive interconnect lines. Ismail and Friedman have derived analytical expressions for calculating the optimum number and size of repeaters [4]. M. Kaur et. al. presented crosstalk analysis using different types of repeaters for coupled MLGNR interconnects at 21 nm technology node [18]. They demonstrated that CNFET repeaters have better OPXT and IPXT delay reduction than FinFET counterparts. Different repeater insertion techniques for bundled SWCNT/MWCNT interconnects have been investigated in [19-20]. The impact of contact resistances on performance of interconnects among the CNTs and metallic electrodes for repeater insertions have been analysed in [21]. They authors of [21] and [22] introduced repeater insertion techniques for optimizing power for RC interconnect models. Zhao et al. analysed optimum repeater insertions for CNT/Cu based nanointerconnects to minimize power consumption and delay considering impact of contact resistance. They have used particle swarm optimization (PSO) method to estimate optimum number of repeaters and their size [23]. The rest of this paper is organized as follows. Section 2 introduces RLC models of Cu/MLGNR interconnects. The repeater insertions are enumerated in Section 3 along with calculation of optimum number and sizing of repeaters. Section 4 discusses about RF analysis of Cu and MLGNR interconnects. Section 5 illustrates crosstalk induced delay and noise results. The comparative results for Cu/MLGNR interconnects using FinFET/CNFET repeaters are covered in Section 6. The conclusions are presented in Section 7. 2. Interconnect Model The Cu resistivity
Details
-
File Typepdf
-
Upload Time-
-
Content LanguagesEnglish
-
Upload UserAnonymous/Not logged-in
-
File Pages23 Page
-
File Size-