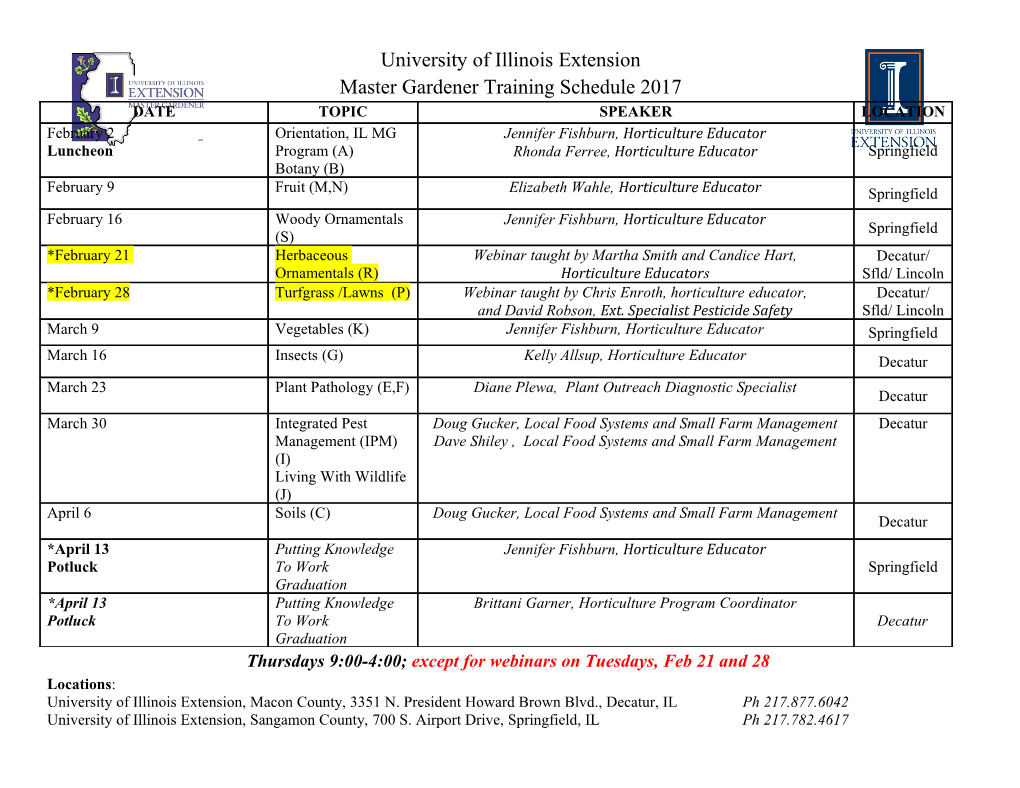
Geosci. Model Dev., 10, 639–671, 2017 www.geosci-model-dev.net/10/639/2017/ doi:10.5194/gmd-10-639-2017 © Author(s) 2017. CC Attribution 3.0 License. Review of the global models used within phase 1 of the Chemistry–Climate Model Initiative (CCMI) Olaf Morgenstern1, Michaela I. Hegglin2, Eugene Rozanov18,5, Fiona M. O’Connor14, N. Luke Abraham17,20, Hideharu Akiyoshi8, Alexander T. Archibald17,20, Slimane Bekki21, Neal Butchart14, Martyn P. Chipperfield16, Makoto Deushi15, Sandip S. Dhomse16, Rolando R. Garcia7, Steven C. Hardiman14, Larry W. Horowitz13, Patrick Jöckel10, Beatrice Josse9, Douglas Kinnison7, Meiyun Lin13,23, Eva Mancini3, Michael E. Manyin12,22, Marion Marchand21, Virginie Marécal9, Martine Michou9, Luke D. Oman12, Giovanni Pitari3, David A. Plummer4, Laura E. Revell5,6, David Saint-Martin9, Robyn Schofield11, Andrea Stenke5, Kane Stone11,a, Kengo Sudo19, Taichu Y. Tanaka15, Simone Tilmes7, Yousuke Yamashita8,b, Kohei Yoshida15, and Guang Zeng1 1National Institute of Water and Atmospheric Research (NIWA), Wellington, New Zealand 2Department of Meteorology, University of Reading, Reading, UK 3Department of Physical and Chemical Sciences, Universitá dell’Aquila, L’Aquila, Italy 4Environment and Climate Change Canada, Montréal, Canada 5Institute for Atmospheric and Climate Science, ETH Zürich (ETHZ), Zürich, Switzerland 6Bodeker Scientific, Christchurch, New Zealand 7National Center for Atmospheric Research (NCAR), Boulder, Colorado, USA 8National Institute of Environmental Studies (NIES), Tsukuba, Japan 9CNRM UMR 3589, Météo-France/CNRS, Toulouse, France 10Institut für Physik der Atmosphäre, Deutsches Zentrum für Luft- und Raumfahrt (DLR), Oberpfaffenhofen, Germany 11School of Earth Sciences, University of Melbourne, Melbourne, Victoria, Australia 12National Aeronautics and Space Administration Goddard Space Flight Center (NASA GSFC), Greenbelt, Maryland, USA 13National Atmospheric and Ocean Administration Geophysical Fluid Dynamics Laboratory (NOAA GFDL), Princeton, New Jersey, USA 14Met Office Hadley Centre (MOHC), Exeter, UK 15Meteorological Research Institute (MRI), Tsukuba, Japan 16School of Earth and Environment, University of Leeds, Leeds, UK 17Department of Chemistry, University of Cambridge, Cambridge, UK 18Physikalisch-Meteorologisches Observatorium Davos – World Radiation Center (PMOD/WRC), Davos, Switzerland 19Graduate School of Environmental Studies, Nagoya University, Nagoya, Japan 20National Centre for Atmospheric Science (NCAS), UK 21LATMOS, Institut Pierre Simon Laplace (IPSL), Paris, France 22Science Systems and Applications, Inc., Lanham, Maryland, USA 23Princeton University Program in Atmospheric and Oceanic Sciences, Princeton, New Jersey, USA anow at: Massachusetts Institute of Technology (MIT), Boston, Massachusetts, USA bnow at: Japan Agency for Marine-Earth Science and Technology (JAMSTEC), Yokohama, Japan Correspondence to: Olaf Morgenstern ([email protected]) Received: 27 July 2016 – Discussion started: 14 September 2016 Revised: 16 December 2016 – Accepted: 9 January 2017 – Published: 13 February 2017 Published by Copernicus Publications on behalf of the European Geosciences Union. 640 O. Morgenstern et al.: CCMI-1 model review Abstract. We present an overview of state-of-the-art ticularly the Chemistry–Climate Model Validation (CCM- chemistry–climate and chemistry transport models that are Val; SPARC, 2010) and the Atmospheric Chemistry and Cli- used within phase 1 of the Chemistry–Climate Model Initia- mate Model Intercomparison Project (ACCMIP; Lamarque tive (CCMI-1). The CCMI aims to conduct a detailed evalua- et al., 2013). These precursors had more limited aims than tion of participating models using process-oriented diagnos- CCMI; in the case of CCMVal, the main aim was to inform tics derived from observations in order to gain confidence in the World Meteorological Organization’s Scientific Assess- the models’ projections of the stratospheric ozone layer, tro- ments of Ozone Depletion (WMO, 2007, 2011, 2015) with pospheric composition, air quality, where applicable global state-of-the-science information about stratospheric ozone climate change, and the interactions between them. Interpre- and its past and projected future evolution. The main out- tation of these diagnostics requires detailed knowledge of the come was SPARC(2010), a comprehensive assessment of radiative, chemical, dynamical, and physical processes incor- the performance of stratospheric CCMs. In the case of AC- porated in the models. Also an understanding of the degree to CMIP, the aim was to inform the 5th Assessment Report which CCMI-1 recommendations for simulations have been of the Intergovernmental Panel on Climate Change (IPCC, followed is necessary to understand model responses to an- 2013) about the roles of “near-term climate forcers”, notably thropogenic and natural forcing and also to explain inter- tropospheric ozone and aerosols, in historical and future model differences. This becomes even more important given climate change. CCMI-1 builds on CCMVal and ACCMIP the ongoing development and the ever-growing complexity by encouraging the participation of coupled atmosphere– of these models. This paper also provides an overview of ocean–stratosphere–troposphere models that represent the the available CCMI-1 simulations with the aim of informing various ways in which stratospheric and tropospheric com- CCMI data users. position are coupled to each other and to the physical cli- mate more consistently than in their predecessor models. A second phase of CCMI, CCMI-2, is planned, where sim- ulations will be conducted jointly with the Aerosol Com- 1 Introduction parisons between Observations and Models (AEROCOM) project and will contribute to the 6th Coupled Model Inter- Climate models have been evolving considerably in recent comparison Project (CMIP6) under the Aerosols and Chem- decades. From relatively simple beginnings, ever more com- istry Model Intercomparison Project (AerChemMIP; Collins ponents have been added, until presently the “Earth System et al., 2016). These will be performed with next-generation Models” (ESMs) define the state of the field. Such models si- models under development and will not be discussed here multaneously serve a variety of purposes including simulat- (http://blogs.reading.ac.uk/ccmi/). ing air quality, tropospheric chemistry, stratospheric ozone, For CCMVal, Morgenstern et al.(2010) described salient and global climate. These applications are strongly coupled; model features of CCMVal-2 models, mostly in tabular e.g. various air pollutants are climate active, stratospheric forms. Their paper builds on numerous publications that de- and tropospheric ozone are coupled in a variety of ways, scribe individual models, or aspects thereof. Here we present and climate change affects atmospheric composition and an update to the tables in Morgenstern et al.(2010), fo- vice versa. Previous-generation models were usually con- cussing in the accompanying text on the changes that have structed for just one of these purposes (e.g. Morgenstern et occurred in the participating models since CCMVal-2. (In all al., 2010; Lamarque et al., 2013). Furthermore, increasingly but three cases, older versions of the CCMI-1 models had biogeochemical feedbacks are considered, e.g. in the form participated in CCMVal-2; see below.) This paper is meant of organic aerosol precursors emitted from land and ocean to support other publications evaluating the CCMI-1 simula- surfaces. However, the additional complexity characterizing tions by providing an overview of the make-up of CCMI-1 ESMs makes these simulations more difficult to evaluate be- models as well as a comprehensive literature list for further cause previously ignored feedbacks now need to be consid- reading. ered. The purpose of this paper is to document the inter- nal make-up of 20 chemistry–climate models (CCMs) and 2 Participating models chemistry-transport models (CTMs) participating in phase 1 of the Chemistry–Climate Model Initiative (CCMI; Eyring There are 20 models participating in CCMI-1 (Tables1 et al., 2013a), a combined activity of the International and2). With three exceptions (CHASER (MIROC-ESM), Global Atmospheric Chemistry (IGAC) and Stratosphere– TOMCAT, MOCAGE), each participating model had a pre- troposphere Processes And their Role in Climate (SPARC) decessor model in CCMVal-2; hence, the focus here will be projects. CCMs are a major stepping stone on the way to- on developments since CCMVal-2. MOCAGE participated wards ESMs, combining physical climate models with an ex- in ACCMIP (Lamarque et al., 2013). Corresponding to the plicit representation of atmospheric chemistry. CCMI-1 con- much broader scope of CCMI, relative to CCMVal-2, salient tinues the legacy of previous CCM inter-comparisons, par- developments in these models include whole-atmosphere Geosci. Model Dev., 10, 639–671, 2017 www.geosci-model-dev.net/10/639/2017/ O. Morgenstern et al.: CCMI-1 model review 641 Table 1. Participating models and contact information. For abbreviations of institution names, see the authors’ affiliations. Model names Institutions Principal investigators (PIs) Email addresses ACCESS CCM U. Melbourne, AAD, K. Stone, [email protected] NIWA R. Schofield, robyn.schofi[email protected] A. Klekociuk, [email protected] D. Karoly, O. Morgenstern CCSRNIES MIROC3.2 NIES H. Akiyoshi, [email protected] Y. Yamashita [email protected] CESM1 CAM4-chem NCAR S. Tilmes, [email protected] J.-F. Lamarque [email protected] CESM1 WACCM NCAR D.
Details
-
File Typepdf
-
Upload Time-
-
Content LanguagesEnglish
-
Upload UserAnonymous/Not logged-in
-
File Pages33 Page
-
File Size-