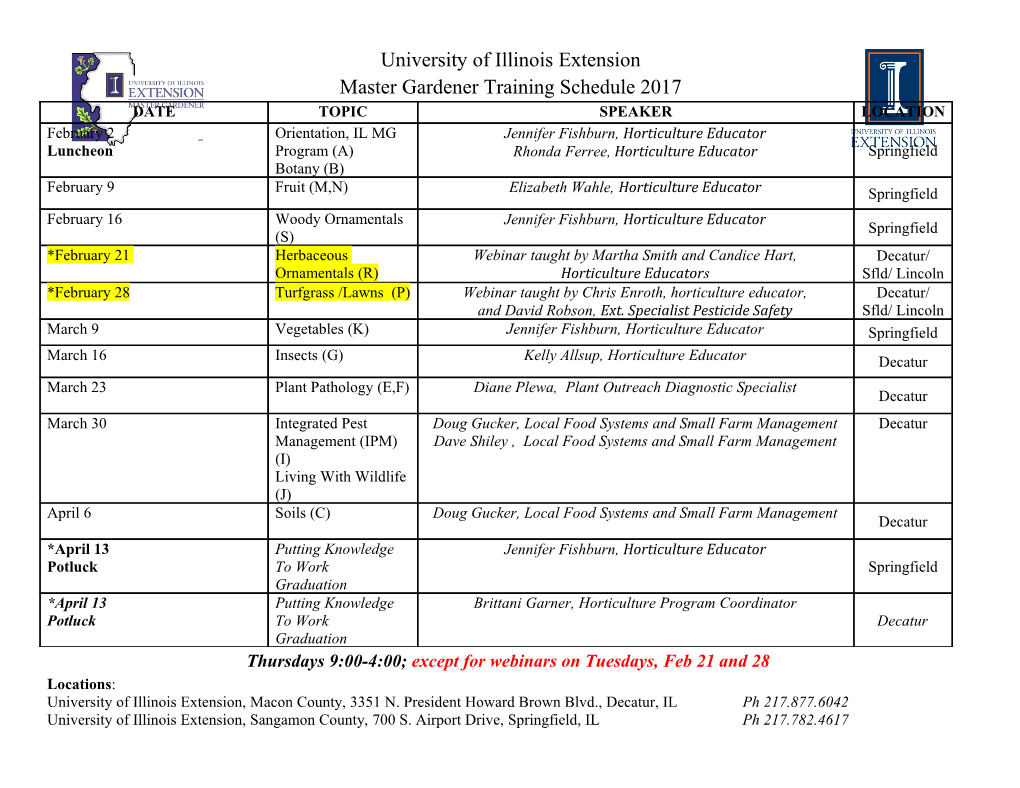
Technological Principles and the Policy Challenges of the Global Positioning System Marlee Chong Spring 2013 Contents 1 Introduction 3 2 History 4 2.1 Location . .4 2.2 LORAN . .5 2.3 GPS Predecessors . .5 2.4 Developing GPS . .6 3 Technology 8 3.1 User Segment . .8 3.2 Control Segment . .8 3.3 Space Segment . .9 3.4 Signal . 10 3.5 Pseudoranging . 11 3.6 Errors and Accuracy . 12 3.6.1 Clock Errors . 12 3.6.2 Atmospheric Errors . 13 3.6.3 System Noise . 13 3.6.4 Multipath Errors . 13 3.6.5 Dilution of Precision . 14 3.6.6 Accuracy . 14 3.7 Vulnerabilities . 15 4 Applications 16 4.1 Military: Smart Bombs . 16 4.2 Positioning: Fault Monitoring . 16 4.3 Navigation: Mobile Phones . 17 4.4 Timing: Stock Exchanges . 17 4.5 Satellites: Nuclear Test Detection . 18 4.6 Signals: Weather Forecasting . 18 5 Policy 19 5.1 Domestic Governance . 19 5.1.1 Defense . 19 1 CONTENTS 2 5.1.2 Civil . 20 5.1.3 Privacy Issues . 21 5.2 Competing Systems . 21 5.2.1 USSR and Russia . 22 5.2.2 European Union . 22 5.2.3 China . 22 5.2.4 Japan . 23 5.2.5 India . 23 5.2.6 International Cooperation . 23 5.3 Modernization . 23 5.3.1 Space Segment . 24 5.3.2 Control Segment . 24 5.3.3 Replacement . 24 5.4 Future and Recommendations . 25 6 Conclusion 26 7 Acknowledgements 27 Chapter 1 Introduction The Global Positioning System (GPS) has become a part of everyday life. In the palm of their hands, users can take advantage of a network of orbiting satellites to determine their coordinates on Earth. However, GPS offers much more than a locator system. Users take advantage of its capabilities for timing, weather forecasting, and even military applications. In fact, GPS was developed during the Cold War through a combination of efforts by the US Navy and US Air Force. Owned and maintained by the United States Government, GPS is available for civilian and commercial use worldwide, free of charge, as a public utility. Still, the US military still has access to encrypted and more accurate signals for defense purposes. GPS has three components: the user segment, control segment, and space segment. The space segment consists of a minimum of 24 satellites, whose orbits are monitored and corrected by the control segment. Receivers in the user segment determine the time it takes the signal to travel from satellites. Despite the seeming ease of pushing a button to use GPS, the details are more convoluted. The system design makes it impossible to actually determine a receiver's position by measuring the time it takes for a satellite's signal to reach the receiver. Instead, a pseudorange is calculated with all of the errors that are implied. Although the system was designed for positioning, navigation, and timing purposes, GPS is also used for other applications. A sample of six applications demonstrate the incredible diversity of uses, some of which are expected while others of which appear to be unrelated. The first four are based on the military, positioning, navigation, and timing applications originally envisioned. The final two appropriate system components for their own purposes. As a dual-use system, GPS is governed by military and civilian agencies and is used for both defense and civil purposes. However, there are issues at the border with regards to privacy. Additional policy concerns come from compet- ing systems, particularly those in development, and the need for international cooperation to coordinate and allow for interoperability. This will remain a concern well into the future with the continued modernization of GPS. 3 Chapter 2 History The development of GPS is part of a long tradition of technologies and meth- ods for navigation. Throughout time, knowing one's location relative to other locations has been important for migration, trade, and the creation of regional boundaries. 2.1 Location Some of the earliest methods of navigation relied on landmarks as reference points. Even today, vestiges of this method remain in the way that we give di- rections, such as the \Left Bank" and \Right Bank" of the Seine River in Paris, and in the determination of borders based on geographic features, such as the Colorado River dividing California and Arizona or the Rio Grande River divid- ing Texas and Mexico. One method used was dead reckoning, which estimates a position based on a known location called a fix, estimated velocity, and elapsed time. However, this method is extremely vulnerable to the accumulation and propagation of errors. If there is a small error in the estimated starting position, velocity, or time, that error will remain in all later assumptions made to determine location using dead reckoning. To minimize the reliance on a small set of reference points to orient locations with each other, a standard frame of reference that system- atically orientated locations was necessary. A geographic coordinate system provided a solution, expanding the possibilities for navigation[22]. Our familiar geographic coordinate system can be traced back to Ancient Greece, where astrologers used latitude and longitude, in addition to time and location of celestial objects, at a person's birth to create natal horoscopes. Al- though latitude could easily be measured on land using the angle above the horizon to Polaris or sun declination and altitude tables, longitude was more difficult to calculate, and navigation in the West using latitude and longitude languished until the mid-fifteenth century. Because the 360 ◦ revolution of the Earth equates to only a 15 ◦ revolution per hour, longitude measurements re- 4 CHAPTER 2. HISTORY 5 quired accurate measurements of time. In fact, two measurements were needed: a fixed reference time, such as Greenwich Mean Time, and a local time[22] . Measuring time accurately required the development of a timepiece that did not need to be stationary. Other challenges included measuring the size of the earth and describing celestial and planetary motion as reference points.These challenges persisted through the 19th century. However, using latitude and lon- gitude to solve the problem of navigation was only one of many methods. Some modern attempts used radio waves, although the need to accurately measure time remained. 2.2 LORAN World War II witnessed the development of several well-known technologies by militaries, from atomic bomb to radar. A lesser-known terrestrial radionavi- gation systems, the Long Range Aid to Navigation (LORAN), was also devel- oped. A master station broadcasted synchronized radio pulses to two or more secondary stations, which then also transmitted the radio waves to the user, who could calculate the time difference between signals based on the known location of the transmitting stations and known emission delays. The time dif- ferences corresponded to hyperbolic lines of position, and the intersection of these defined the user's two-dimensional position. While the 1960s saw global coverage with the launch of the Omega system, improvements continued to be made to LORAN[18, p. 123-127]. Its successor, Loran-C, remained in operation until 2010 and could be integrated with GPS or used as a backup system, espe- cially in areas where incoming satellite signals where blocked[14, Termination of LORAN-C]. 2.3 GPS Predecessors The 1957 launch of Sputnik by the USSR not only transformed the space race into a race to put a man on the moon but also opened up possibilities for the applications of satellites. At John Hopkins University's Applied Physics Laboratory (APL), physicists noticed a measurable Doppler shift in the satellite signal. Dr. Frank T. McClure realized that these measurements could be used to provide the location of a stationary observer. In 1960, physicist and president of the Aerospace Corporation Ivan Getting proposed that satellites could not only provide information about position but also improve navigation by land forces. That same year, APL developed a prototype satellite positioning system for the US Navy. By 1972, this functioned as the Navy's space-based navigation system called the Navigation Satellite System (NAVSAT, also known as Transit), which operated until 1996. The USSR developed Cicada, a system based on the same principles, but these systems were limited by the need to have an unobstructed line of sight due to the short wavelengths. Furthermore, the processing time and accuracy, while suitable for the needs of slow-moving naval vessels, were CHAPTER 2. HISTORY 6 insufficient for aircraft[23, p. 2]. Thus, the US Air Force had been investigating a competing idea, and together, their efforts led to the development of GPS. Transit was only the first of three important predecessors to GPS. The second was Timed Navigation (Timation), a program by Roger Easton at the Naval Re- search laboratory that improved the precision of clocks and ultimately brought atomic clocks to space. These stable space-based clocks improved the accuracy of timing information because like earlier terrestrial positioning methods, satel- lite positioning requires highly accurate timepieces to determine location. To determine the user's location, Timation used Side Tone Ranging, where a satel- lite broadcast a clear and obvious signal that could easily be jammed. However, each satellite would need to broadcast on a fundamentally different frequency [24]. The US Air Force's 621B program was the third competing design. A satel- lite positioning system designed to offer three-dimensional coverage and global service, 621B used digital signals. This meant that all of the satellites could share a spread spectrum signal, differing only due to Doppler shifts and avoiding inter-channel bias shifts. 621b also explored variations on number and configu- ration of satellites for a network providing global coverage[20].
Details
-
File Typepdf
-
Upload Time-
-
Content LanguagesEnglish
-
Upload UserAnonymous/Not logged-in
-
File Pages31 Page
-
File Size-