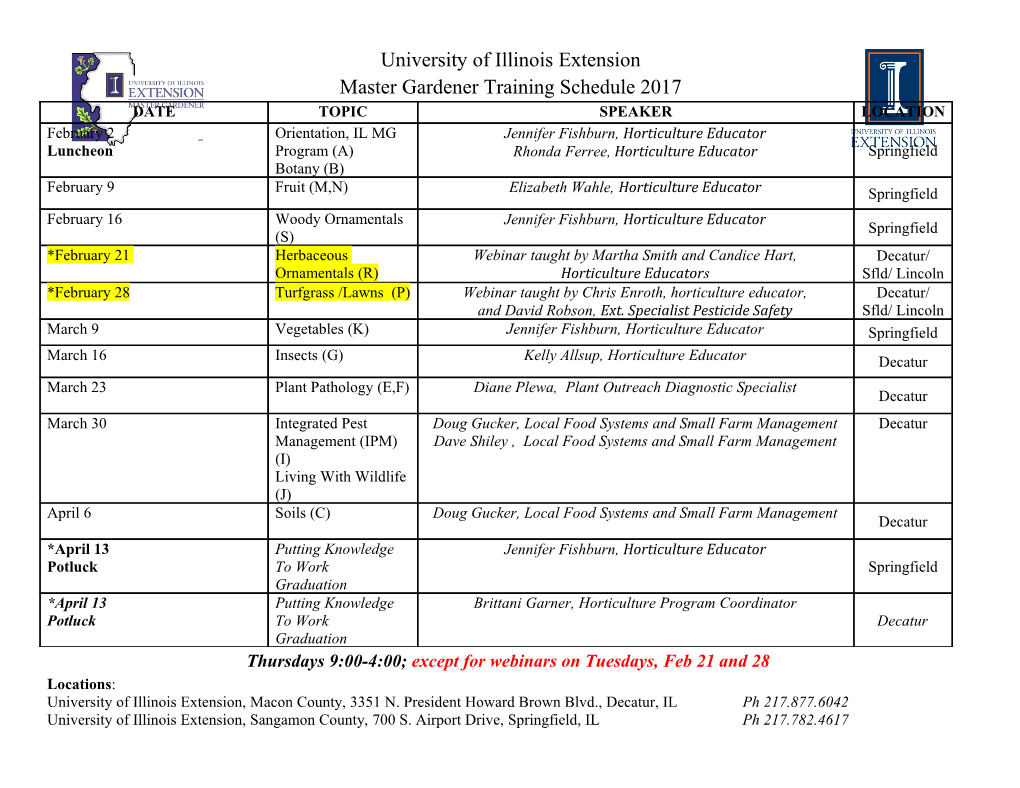
E-Journal of Advanced Maintenance Vol.9-2 (2017) 138-144 Japan Society of Maintenology Why graphite ? A Structural Study from the Atomic to the Macrocsopic scales. Zhou ZHOU1*, Wim BOUWMAN1, Henk SCHUT1, Catherine PAPPAS1 1 Department of Radiation Science and Technology, Delft University of Technology, Mekelweg 15, 2629 JB Delft, The Netherlands ABSTRACT Graphite is an important moderator material in nuclear reactors, which has a complex structure, where complexity refers to two main respects: defects and disorder within the crystallites, and porosity including cracks and pores with lengths varying over six orders of magnitude, from nanometers to millimeters. A comprehensive characterization of the structure, needed e.g. to predict structural changes for long-term safe operation of nuclear reactors, requires the complementary input from several techniques to cover the relevant length scale range. In this work X-ray/neutron diffraction, small angle neutron scattering (SANS), spin echo SANS (SESANS) and neutron imaging have been applied to investigate the structure of nuclear graphite from atomic to macroscopic length scales: from nm to mm. The results provide a key to model and quantify disorder at the atomic level. At the meso- and macro-scopic levels a fractal structure is found that spans over an extraordinary large scale of lengths of 6 orders of magnitude and has fractal dimensions close to 2.5, a case where surface and mass fractal dimensions coincide. KEYWORDS ARTICLE INFORMATION graphite, diffraction, neutron irradiation, structure characterization, Article history: neutron scattering, neutron radiography, stacking fault, fractal Received 7 November 2016 Accepted 27 April 2017 1. Introduction Since Chicago Pile 1 in 1942, graphite has been used as a neutron moderator and structural component in several types of nuclear reactors. More recently, it has been considered as the material of choice for the next generation Very High Temperature Reactor (VHTR). During operation of nuclear reactors, graphite undergoes significant dimensional, physical and mechanical properties changes due to the irradiation damage. It is thus important to investigate these changes and link them to the structure. The ultimate goal is to be able to predict the lifetime of graphite under irradiation as this is closely related to the safe operations of nuclear reactors [1]. Structural investigations often involve X-ray diffraction (XRD) and scanning/transmission electron microscopy (SEM/TEM). XRD is indeed the most common method for studying atomic structures. However, the correct interpretation of XRD patterns from graphite samples is not trivial because of (a) the inherent disorder, which can significantly affect the shape of the diffracted peaks, and (b) the high penetration depth of X-rays. Electron microscopy enables the investigation on the defects and porous structure. However, it probes only surface layers and does not provide a quantitative description of the bulk material. Therefore, the results may not be directly relevant to the bulk properties e.g. of a graphite block inside a nuclear reactor [2]. In this work, the crystal structure of nuclear graphite has been investigated by both X-ray and neutron diffraction [3]. The diffraction patterns have been analyzed using a structural model, which has been developed specifically for graphite and quantifies the inherent disorder of the atomic structure. In addition, the structure of the pores has been studied explored by combining small angle neutron scattering (SANS), spin-echo SANS (SESANS) and neutron imaging [4]. The combination of these techniques provides structural information over six orders of magnitude in length, from * Corresponding author, E-mail: [email protected] ISSN-1883-9894/10 © 2010 – JSM and the authors. All rights reserved. 138 Z. Zhou, et al./ Title of the Paper nanometers to millimeters, which is needed in order to understand the graphite structure at the meso- and macro-scopic levels. The ultimate goal would be to establish a direct link between the changes in structure and the macroscopic behavior of nuclear graphite. 2. Experimental 2.1 Graphite samples Five different types of non-irradiated graphites have been investigated including four nuclear grade graphites: PGA (used in the early gas-cooled Magnox reactors in UK), PCEA (a candidate for the very high temperature reactor in USA), IG110 (currently used in HTGR reactors in Japan) and RID (used at the research reactor of the Reactor Institute Delft), as well as a non-nuclear graphite 1940 PT, which is a high-density graphite manufactured for refractory applications. Irradiated graphite samples were provided by the graphite irradiation program INNOGRAPH-1c conducted by the Nuclear Research and Consultancy Group (NRG) in the High Flux Reactor (HFR) in Petten, The Netherlands. The samples were irradiated at a high temperature of 750 ºC with low doses (~1.5 and ~2.2 dpa) and for confidentiality reasons they are kept anonymous in this work [5]. 2.2 Methods XRD was performed on a lab Panalytical X’pert Pro diffractometer with Cu anode (45 kV, 40 mA, λ = 1.54187 Å). Neutron diffraction was performed on 3T2, a high-resolution powder diffractometer in Laboratoire Léon Brillouin (LLB), Saclay, France, with an incident neutron wavelength of λ = 1.225 Å. SANS was performed on two spectrometers PAXE and TPA in LLB. The measurements on PAXE were done with λ = 0.37, 0.6, and 1.7 nm, respectively, covering the Q range 3×10-2 ≤ Q ≤ 5 nm-1 and on TPA with λ = 0.6 nm, covering the Q range 6×10-3 ≤ Q ≤ 1×10-1 nm-1. SESANS was performed on the dedicated instrument of the Reactor Institute Delft at λ = 0.205 nm, with Δλ/λ = 5% and covered spin-echo length scales from 30 nm to 20 μm. The neutron imaging measurements took place on the cold neutron facility, ICON, of the Paul Scherrer Institute, Switzerland. The samples were placed as close as possible to the detector and the spatial resolution of the images reached ~30 μm. 3. Results and discussion 3.1 X-ray and neutron diffraction Fig. 1(a) displays the measured XRD pattern from a virgin IG110 graphite sample (black circles), which is hardly reproduced by the standard Rietveld method and the program GSAS (blue line). This is because the inherent disorder, such as the presence of local ABC stacking, the random shift between adjacent layers and the anisotropic crystallite size cannot be accounted for by a standard Rietveld refinement. In order to overcome these limitations, it is important to use a model that incorporates the specific structural particularities of graphite, and includes disorder. Such a refinement program is CARBONXS, that is based on a model developed by Shi, et al [6]. The model introduces interlayer spacing fluctuations, characterized by the standard deviation σ around the average value <d002> besides the average lattice constants <a> and <d002>. It also includes two different characteristic lengths, one along the c-axis Lc and one in the plane La, to quantify the volumes that scatter coherently neutron or X-ray beams. In addition, the model quantifies the stacking disorder through two parameters: PRS, the probability for finding a random shift between adjacent layers; and P3R, the probability for finding a local ABC stacking sequence. In addition, the program CARBONXS also includes corrections for the penetration depth of X-rays, the X-ray polarization, the Lorentz factor, a preferred sample orientation and an isotropic Debye-Waller factor. The red dash line in Fig. 1(a) represents the fit of the measured XRD pattern by CARBONXS, and illustrates a major improvement as compared with the fit by GSAS. The resulting refinements of all graphites show that <d002> is an essential indicator of the perfection and graphitization degree of the structure. The smaller <d002> implies larger coherent lengths Lc and La, which quantify the extension of the coherent regions and thus the order of the microstructure. The value of <d002> for nuclear graphites is close to 3.360 Å, which is slightly larger than the value for a perfect graphite crystal that amounts to 3.354 Å. This implies that nuclear graphites are highly disordered, despite their high degree of purity and the fact that they are manufactured in a very high temperature (2600 ̵ 3000 ºC). In addition, the fits lead to 15-25% random shift PRS and about 7% 139 Z. Zhou, et al./ Title of the Paper ABC stacking faults P3R, the standard deviation of interlayer spacing fluctuations σ≫<d002>-3.354, indicating other disorder in the graphite structure, such as dislocations, crosslinks, vacancies and interstitial defects. Fig. 1. Measured and calculated X-ray diffraction patterns in log-lin scale for (a) a non-irradiated IG110 graphite sample, and (b) a low dose (2.2 dpa), high temperature (750°C) neutron irradiated graphite sample. The red lines indicate the refinements based on the program CARBONX and the blue line the standard Rietveld refinement using the program GSAS. The neutron irradiation effects on graphite structure have been investigated in the same way [7]. Fig. 1(b) depicts the measured XRD pattern of a neutron irradiated (750 ºC, 2.2 dpa) graphite sample from INNOGRAPH and the fit by CARBONXS. The refinement shows that irradiation does not affect the lattice constant <a> but increases <d002> by ~0.5%, which seems to approache saturation at ~2.2 dpa. The increase of <d002> is attributed to interstitial faults caused by atom displacements during irradiation. Furthermore, neutron irradiation deteriorates the stacking of layers within the coherent regions as indicated by the increase of PRS and the decrease of P3R. In addition, La decreases continuously up to a dose of ~1.5 dpa, indicating induced in-plane defects, as well as the breaking, bending and displacement of the basal planes. On the other hand, the relative changes of <d002> and Lc up to a dose of ~1.5 dpa indicate that, along the c-axis, the number of layers in the coherent regions slightly increases, which may be due to (1) the formation of interstitial dislocation loops and/or (2) the closing of micro-cracks between slightly disoriented coherent regions.
Details
-
File Typepdf
-
Upload Time-
-
Content LanguagesEnglish
-
Upload UserAnonymous/Not logged-in
-
File Pages7 Page
-
File Size-