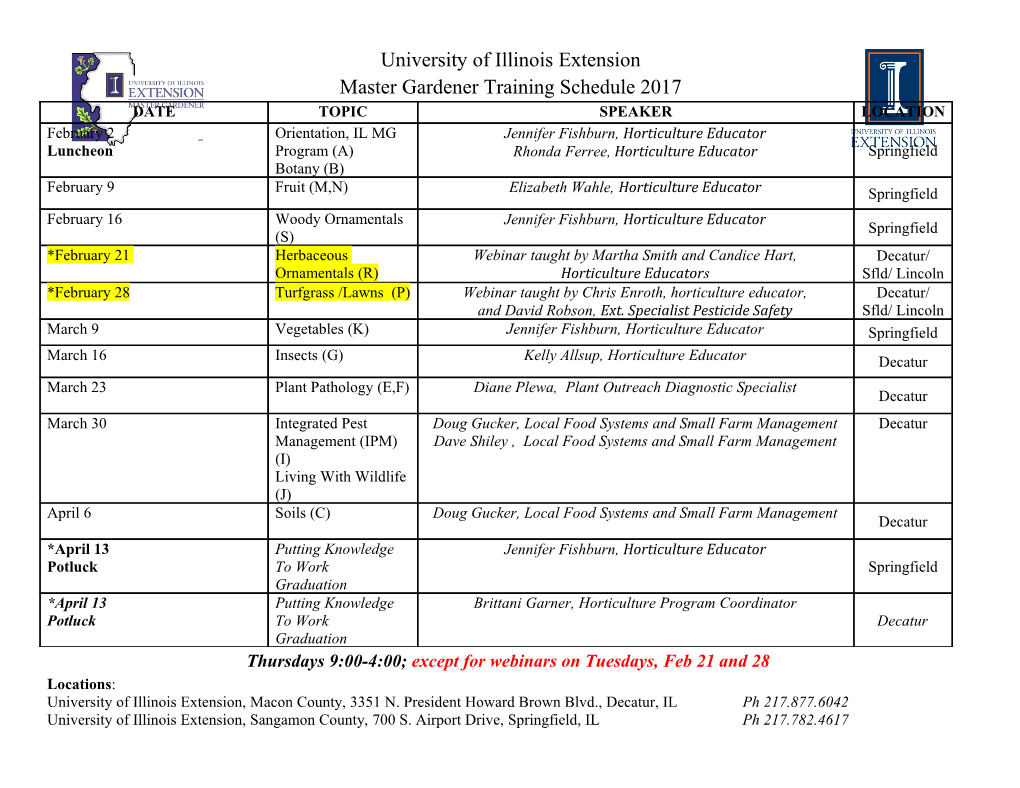
Metaproteomics of a gutless marine worm and its PNAS PLUS symbiotic microbial community reveal unusual pathways for carbon and energy use Manuel Kleinera,b,1, Cecilia Wentrupa, Christian Lotta,c, Hanno Teelinga, Silke Wetzela, Jacque Youngd,e, Yun-Juan Changd, Manesh Shahd, Nathan C. VerBerkmoesd, Jan Zarzyckif, Georg Fuchsf, Stephanie Markertg, Kristina Hempelb, Birgit Voigtb, Dörte Becherb, Manuel Liebekeh,i, Michael Lalkh, Dirk Albrechtb, Michael Heckerb,g, Thomas Schwederg,h,1, and Nicole Dubiliera,1 aSymbiosis Group, Max Planck Institute for Marine Microbiology, 28359 Bremen, Germany; bInstitute for Microbiology and hInstitute of Pharmacy, University of Greifswald, 17487 Greifswald, Germany; cElba Field Station, HYDRA Institute for Marine Sciences, Località Fetovaia, 57034 Campo nell’Elba, Italy; dOak Ridge National Laboratory, Chemical Science Division, Oak Ridge, TN 37831; eGraduate School for Genome Science and Technology, University of Tennessee, Knoxville, TN 37996; fDepartment of Microbiology, Faculty of Biology, University of Freiburg, 79104 Freiburg, Germany; gInstitute of Marine Biotechnology, 17489 Greifswald, Germany; and iBiomolecular Medicine, Department of Surgery and Cancer, Faculty of Medicine, Imperial College London, London, United Kingdom SW7 2AZ Edited by Charles R. Fisher, Pennsylvania State University, University Park, PA, and accepted by the Editorial Board February 29, 2012 (received for review December 22, 2011) Low nutrient and energy availability has led to the evolution of pounds. Chemosynthetic symbioses thus are able to thrive in numerous strategies for overcoming these limitations, of which habitats where organic carbon sources are rare, such as the deep symbiotic associations represent a key mechanism. Particularly sea, and the symbionts often are so efficient at providing nutri- striking are the associations between chemosynthetic bacteria and tion that many hosts have reduced their digestive systems (4). marine animals that thrive in nutrient-poor environments such as The marine oligochaete Olavius algarvensis is a particularly the deep sea because the symbionts allow their hosts to grow on extreme example of a nutritional symbiosis: These worms are fi inorganic energy and carbon sources such as sul de and CO2. Re- dependent on their chemosynthetic symbionts for both their nu- MICROBIOLOGY markably little is known about the physiological strategies that trition and their excretion, because they have reduced their enable chemosynthetic symbioses to colonize oligotrophic envi- mouth, gut, and nephridial excretory organs completely (5). O. ronments. In this study, we used metaproteomics and metabolo- algarvensis lives in coarse-grained coastal sediments off the island mics to investigate the intricate network of metabolic interactions of Elba, Italy, and migrates between the upper oxidized and the in the chemosynthetic association between Olavius algarvensis, lower reduced sediment layers (6). It hosts a stable and specific a gutless marine worm, and its bacterial symbionts. We propose microbial consortium consisting of five bacterial endosymbionts previously undescribed pathways for coping with energy and nu- in its body wall: two aerobic or denitrifying gammaproteobacterial trient limitation, some of which may be widespread in both free- sulfur oxidizers (γ1- and γ3-symbionts), two anaerobic deltapro- living and symbiotic bacteria. These pathways include (i) a path- teobacterial sulfate reducers (δ1- and δ4-symbionts), and a spi- way for symbiont assimilation of the host waste products acetate, rochete with an unknown metabolism (7, 8). The sulfate-reducing propionate, succinate and malate; (ii) the potential use of carbon δ-symbionts provide the sulfur-oxidizing γ-symbionts with re- monoxide as an energy source, a substrate previously not known duced sulfur compounds as an internal energy source for auto- to play a role in marine invertebrate symbioses; (iii) the potential trophic CO fixation via the Calvin–Benson cycle, thus explaining use of hydrogen as an energy source; (iv) the strong expression of 2 how O. algarvensis can thrive in its sulfide-poor environment (6, high-affinity uptake transporters; and (v) as yet undescribed en- 9). As in all living organisms, the symbiosis is dependent on ex- ergy-efficient steps in CO fixation and sulfate reduction. The high 2 ternal energy sources, but to date the identity of these sources has expression of proteins involved in pathways for energy and car- remained unclear. bon uptake and conservation in the O. algarvensis symbiosis indi- Like the vast majority of symbiotic microbes, the O. algarvensis cates that the oligotrophic nature of its environment exerted a fi strong selective pressure in shaping these associations. symbionts have de ed cultivation attempts, making cultivation- independent techniques essential for their analysis. A meta- 3-hydroxypropionate bi-cycle | Calvin cycle | proton-translocating genomic analysis of the O. algarvensis symbionts yielded initial pyrophosphatase | pyrophosphate dependent phosphofructokinase | insights into their potential metabolism (9), but the incomplete metagenomics genome sequences hindered the reconstruction of complete met- abolic pathways, leaving many questions unanswered (10). Fur- rowth in nutrient-limited environments presents numerous Gchallenges to organisms. Symbiotic and syntrophic relation- ships have evolved as particularly successful strategies for coping with these challenges. Such nutritional symbioses are widespread Author contributions: M.K., G.F., B.V., D.B., M. Liebeke, M. Lalk, M.H., and T.S. designed research; M.K., C.W., C.L., H.T., S.W., J.Y., Y.-J.C., N.C.V., J.Z., K.H., M. Liebeke, and D.A. in nature and, for example, have enabled plants to colonize ni- performed research; N.C.V., G.F., M. Lalk, M.H., T.S., and N.D. contributed new reagents/ trogen-poor soils and animals to thrive on food sources that lack analytic tools; M.K., C.W., J.Y., M.S., N.C.V., J.Z., S.M., B.V., D.B., M. Liebeke, and N.D. essential amino acids and vitamins (1). Chemosynthetic symbi- analyzed data; and M.K. and N.D. wrote the paper. oses, discovered only 35 years ago at hydrothermal vents in the The authors declare no conflict of interest. deep sea, revolutionized our understanding of nutritional asso- This article is a PNAS Direct Submission. C.R.F. is a guest editor invited by the Editorial Board. ciations, because these symbioses enable animals to live on in- Freely available online through the PNAS open access option. organic energy and carbon sources such as sulfide and CO2 (2, 3). 1To whom correspondence may be addressed. E-mail: [email protected], The chemosynthetic symbionts use the energy obtained from [email protected], or [email protected]. fi fi oxidizing reduced inorganic compounds such as sul de to x This article contains supporting information online at www.pnas.org/lookup/suppl/doi:10. CO2, ultimately providing their hosts with organic carbon com- 1073/pnas.1121198109/-/DCSupplemental. www.pnas.org/cgi/doi/10.1073/pnas.1121198109 PNAS Early Edition | 1of10 Downloaded by guest on October 1, 2021 thermore, as in all genomic analyses, detailed insights into the physiology and metabolism of an organism are limited, because these analyses can predict only the metabolic potential of an or- ganism, not its actual metabolism and physiology (11). This lim- itation is most apparent in a multimember community in which the interactions between the different members and between these members and their environment lead to a level of metabolic complexity that can greatly exceed the predictive ability of genomic reconstructions from single species. Although metagenomic analyses reveal the metabolic poten- tial of a microbial community, metaproteomic and metabolomic analyses provide evidence for the metabolic and physiological processes that actually are used by the community. In this study, we used metaproteomics and metabolomics as well as enzyme assays and in situ analyses of potential energy sources to gain an in-depth understanding of the intricate interactions between O. algarvensis and its microbial symbiont community and between the members of this community and their environment. Our goal was to identify the compounds that provide energy for the Fig. 1. Positive effect of symbiont enrichment using density-gradient cen- symbiosis, the functional roles of the different partners, and their trifugation. Enrichment considerably increased the number of identified interactions within the symbiosis. proteins from a given symbiont compared with analyses of whole worms. Average protein numbers were calculated for 2D LC-MS/MS experiments (SI γ δ Results and Discussion Appendix, Table S1). n = 2 for 1-symbiont enrichments; n = 2 for 1-sym- biont enrichments; and n = 4 for whole-worm samples. Both metagenomic High Coverage of the Symbiosis Metaproteome and Metabolome. We and proteomic binning information was used for assignment of proteins to identified and quantified a total of 2,819 proteins and 97 a symbiont. metabolites in O. algarvensis and its symbiotic community (SI Appendix, Tables S1 and S2 and Datasets S1 and S2) using dif- ferent methods for both the metaproteomic and the metab- teomic analyses are consistent with this model of syntrophic olomic analyses to overcome the intrinsic biases inherent in sulfur cycling, with abundantly expressed sulfur oxidation pro- a single detection method (SI Appendix, SI Text). For host pro- teins detected in the γ-symbionts and sulfate reduction proteins
Details
-
File Typepdf
-
Upload Time-
-
Content LanguagesEnglish
-
Upload UserAnonymous/Not logged-in
-
File Pages10 Page
-
File Size-