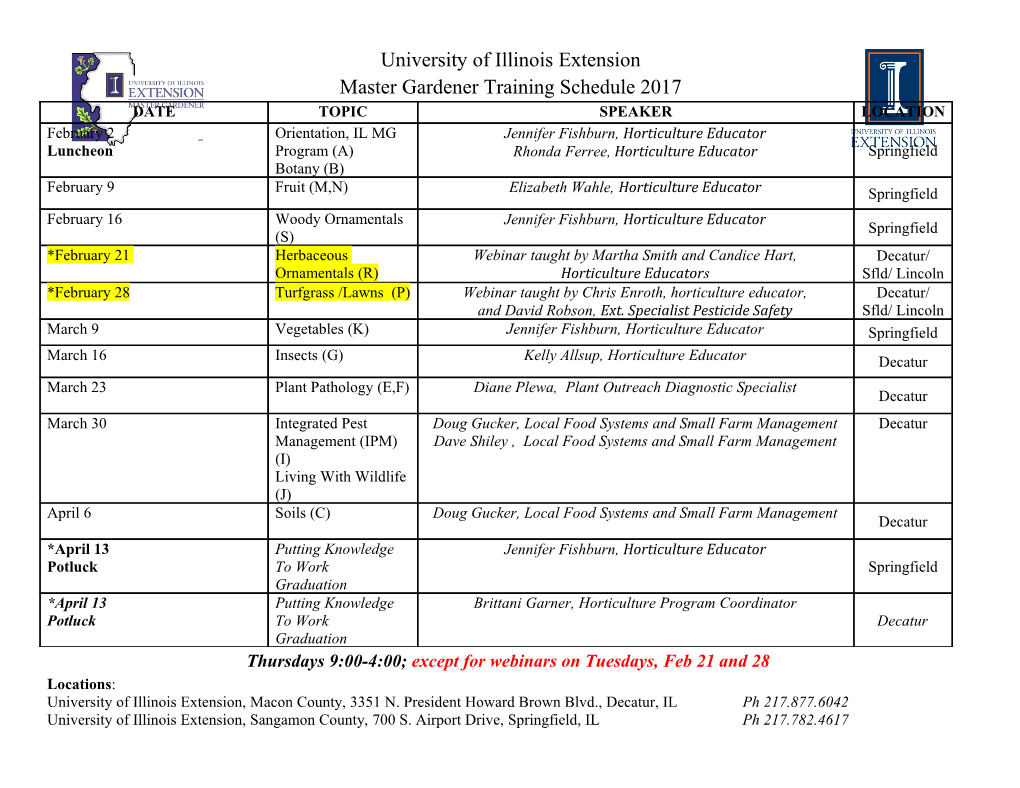
Theoretical Investigations into the Effects of Reaction Environment on Acid-Catalyzed Dehydration of Biomass-Derived Oxygenates A DISSERTATION SUBMITTED TO THE FACULTY OF THE UNIVERSITY OF MINNESOTA BY Chotitath Sanpitakseree IN PARTIAL FULFILLMENT OF THE REQUIREMENTS FOR THE DEGREE OF DOCTOR OF PHILOSOPHY Advised by Prof. Matthew Neurock April 2020 Copyright © 2020 by Chotitath Sanpitakseree Abstract The growing concerns over greenhouse gas emissions and the limited fossil fuel resources has driven the development of alternative carbon-neutral energy sources. Lignocellulosic biomass is considered an attractive option as they are renewable and are available in abundance at low cost. However, these feedstocks possess relatively low carbon/oxygen ratio and thus making the oxygen removal necessary to increase the energy density. The chemical treatment of biomass in aqueous phase typically involves initial decomposition of sugar polymers into individual sugar monomers followed by a series of acid-catalyzed dehydration steps. The intermediate products that from include 5- hydroxymethylfurfural (HMF), 2-furfuraldehyde (furfural), levulinic acid, and formic acid which can be further upgraded to liquid fuel. These processes are relatively cost-efficient as they can be carried out in aqueous phase at low temperature. Despite its economic potential, very little is known about the effects of solvent on the dehydration kinetics. In this work, we utilized ab initio quantum chemical methods to examine the underlying principles that control the reactivity and selectivity of biomass conversion reactions, especially Brønsted acid-catalyzed dehydration of biomass-derived oxygenates in organic solvent/water mixtures. Recent experimental studies have shown that the rate of acid-catalyzed dehydration of sugars increases by up to two orders of magnitude when the reaction is carried out in polar aprotic solvent/water mixtures such as DMSO/water mixtures and acetonitrile/water mixtures in comparison to water solvent. Furthermore, the addition of metal halides such as chloride anion into the reaction media has been shown to significantly improve the i reactivity and selectivity towards the desired HMF product. In order to develop better understandings of these observations, we used ab initio molecular dynamics (AIMD) together with classical molecular dynamics simulations to explore the effects of reaction environment in liquid phase on the dehydration reactions of biomass-derived oxygenates. Our calculations suggest that the rate of Brønsted acid catalyzed dehydration in polar aprotic solvent/water mixtures is governed by the relative stability between reactants in comparison to transition state in the rate determining step. This difference in the stability is in turn controlled by the different extent of solvation for these reactive species in different solvents which explains the reactivity trend observed experimentally. The molecular dynamics trajectories for organic solvent/water systems reveal that the solvent- solute as well as solvent-solvent interactions result in the reorganization of solvent molecules around the solvated species, leading to the formation of hydrophilic domain on the reactive polyol hydroxyl groups in which the acidic proton and other hydrophilic species reside. The localization of these reactive species in close vicinity facilitates the dehydration reaction, stabilizes the charged transition state, and lowers the activation free energy of dehydration. The investigation is also extended to different reaction paths leading to the formation of humins side products to elucidate the effects of solvent on the selectivity of dehydration reactions toward HMF. In the second part of this thesis, we focus on the use of heterogeneous catalyst for dehydration reactions. The use of heterogeneous catalyst is favored over homogeneous catalyst due to the ease of catalyst recovery. However, the ab initio computational investigation of liquid-phase reactions in microporous catalyst is challenging as the result ii can be sensitive to the number of solvent molecules and reactive species available in the zeolite pore. In this work, we thus focused on developing an atomistic model to predict the adsorption properties of water molecules in industrially common Faujasite and Mordenite zeolites. The model features a new ‘cluster swap’ Monte Carlo move that allows simultaneous simulation of cation siting and aluminum distribution within the zeolite framework. The simulation results were found to satisfactorily reproduce the cationic siting in Faujasite as well as aluminum occupancy of the framework T-sites in Mordenite over a broad range of Si/Al ratios. The obtained parameters can be further improved to include the adsorption affinity for each solvent and solvated species. iii Table of Contents Abstract ............................................................................................................................... i Table of Contents ............................................................................................................. iv List of Tables ................................................................................................................... vii List of Figures ................................................................................................................. viii Chapter 1 – Introduction ................................................................................................. 1 Chapter 2 – Solvent-Enabled Control of Reactivity for Liquid Phase Reactions of Biomass-Derived Compounds .......................................................................................... 6 2.1 Introduction ............................................................................................................... 6 2.2 Results and Discussions ............................................................................................ 7 2.2.1 Experimental reaction kinetics studies ............................................................... 7 2.2.2 Molecular dynamics simulations ...................................................................... 12 2.2.3 Solvent-enabled control of reactivity for liquid-phase reactions ..................... 21 2.3 Conclusions ............................................................................................................. 23 2.4 Methods ................................................................................................................... 24 2.4.1 Reaction kinetics experiments .......................................................................... 24 2.4.2 Reaction mechanisms ....................................................................................... 28 2.4.3 Simulation details ............................................................................................. 28 2.4.4 Free energy calculations .................................................................................. 30 2.4.5 Radial distribution functions ............................................................................ 34 2.5 Supplementary Information ..................................................................................... 35 Chapter 3 – Effects of Chloride Ions in Acid-Catalyzed Biomass Dehydration Reactions in Polar Aprotic Solvents .............................................................................. 70 3.1 Introduction ............................................................................................................. 70 3.2 Experimental Results ............................................................................................... 71 3.3 Discussion ............................................................................................................... 75 3.3.1 Experimental discussion ................................................................................... 75 3.2.2 Computational discussion ................................................................................ 85 3.4 Conclusions ............................................................................................................. 95 3.5 Methods ................................................................................................................... 95 iv 3.5.1 Reaction kinetics experiments .......................................................................... 95 3.5.2 NMR spectroscopy ............................................................................................ 97 3.5.3 Solution calorimetry ......................................................................................... 98 3.5.4 Computational simulations ............................................................................... 99 3.6 Supplementary Information ................................................................................... 105 Chapter 4 – Theoretical Investigation of Solvent and Chloride Effects on the Production 5-Hydroxymethylfurfural from Glucose ................................................ 129 4.1 Introduction ........................................................................................................... 129 4.2 Methods ................................................................................................................. 131 4.2.1 Reaction coordinates for glucose isomerization ............................................ 134 4.2.2 Reaction coordinates for fructose dehydration .............................................. 135 4.2.3 Reaction coordinates for glucose
Details
-
File Typepdf
-
Upload Time-
-
Content LanguagesEnglish
-
Upload UserAnonymous/Not logged-in
-
File Pages270 Page
-
File Size-