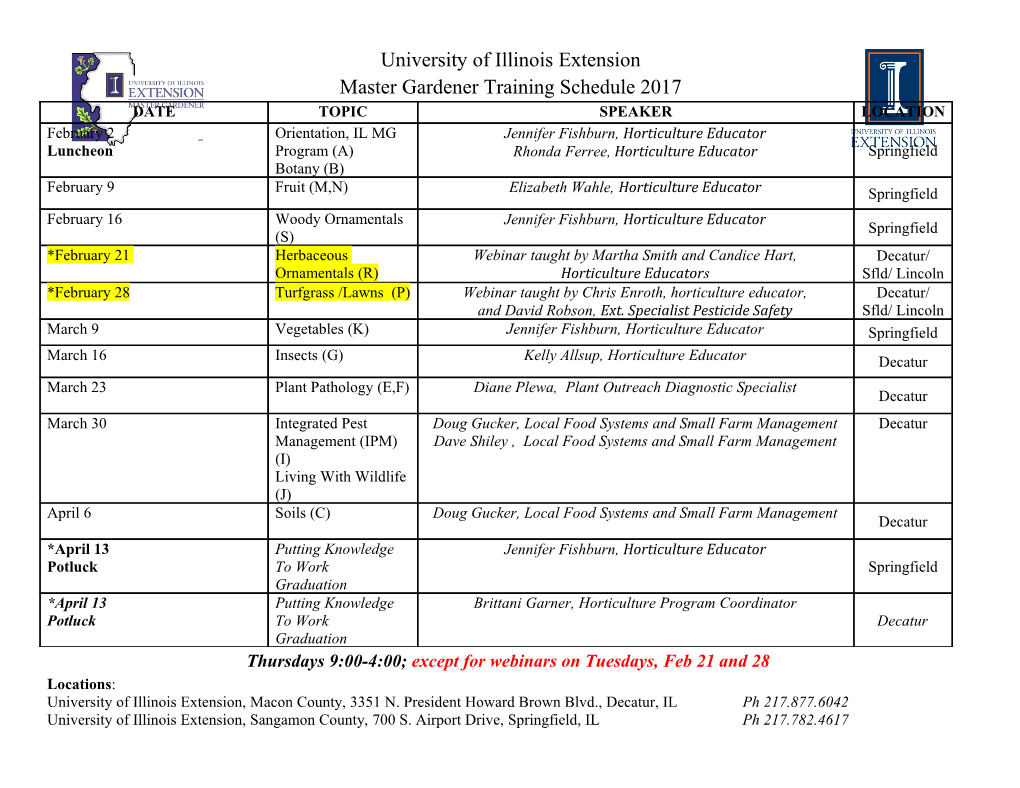
JOURNAL OF GEOPHYSICAL RESEARCH, VOL. 111, XXXXXX, doi:10.1029/2005JE002510, 2006 Click Here for Full Article 2 Identification of volcanic rootless cones, ice mounds, and impact 3 craters on Earth and Mars: Using spatial distribution as a remote 4 sensing tool 1 1 1 2 3 5 B. C. Bruno, S. A. Fagents, C. W. Hamilton, D. M. Burr, and S. M. Baloga 6 Received 16 June 2005; revised 29 March 2006; accepted 10 April 2006; published XX Month 2006. 7 [1] This study aims to quantify the spatial distribution of terrestrial volcanic rootless 8 cones and ice mounds for the purpose of identifying analogous Martian features. Using a 9 nearest neighbor (NN) methodology, we use the statistics R (ratio of the mean NN distance 10 to that expected from a random distribution) and c (a measure of departure from 11 randomness). We interpret R as a measure of clustering and as a diagnostic for 12 discriminating feature types. All terrestrial groups of rootless cones and ice mounds are 13 clustered (R: 0.51–0.94) relative to a random distribution. Applying this same 14 methodology to Martian feature fields of unknown origin similarly yields R of 0.57–0.93, 15 indicating that their spatial distributions are consistent with both ice mound or rootless 16 cone origins, but not impact craters. Each Martian impact crater group has R 1.00 (i.e., 17 the craters are spaced at least as far apart as expected at random). Similar degrees of 18 clustering preclude discrimination between rootless cones and ice mounds based solely on 19 R values. However, the distribution of pairwise NN distances in each feature field 20 shows marked differences between these two feature types in skewness and kurtosis. 21 Terrestrial ice mounds (skewness: 1.17–1.99, kurtosis: 0.80–4.91) tend to have more 22 skewed and leptokurtic distributions than those of rootless cones (skewness: 0.54–1.35, 23 kurtosis: À0.53–1.13). Thus NN analysis can be a powerful tool for distinguishing 24 geological features such as rootless cones, ice mounds, and impact craters, particularly 25 when degradation or modification precludes identification based on morphology alone. 26 Citation: Bruno, B. C., S. A. Fagents, C. W. Hamilton, D. M. Burr, and S. M. Baloga (2006), Identification of volcanic rootless 27 cones, ice mounds, and impact craters on Earth and Mars: Using spatial distribution as a remote sensing tool, J. Geophys. Res., 111, 28 XXXXXX, doi:10.1029/2005JE002510. 30 1. Introduction Mark, 1987; Cabrol et al., 2000; Chapman and Tanaka, 46 2001]. Water-rich localities on Mars not only represent 47 31 [2] The objective of this research is to refine and apply potential niches for past biological development, but they 48 32 the nearest neighbor (NN) technique used by Bruno et al. are also prospective sources of water for future Mars 49 33 [2004] to distinguish among different origins for small missions. 50 34 (less than a few km), circular to elongate, positive-relief [3] Rootless volcanic cones are an important diagnostic 51 35 mounds and cones, for the purposes of understanding the of ice distribution in the near-surface regolith [Greeley and 52 36 local geology and, ultimately, constructing a historical Fagents, 2001; Lanagan et al., 2001; Fagents et al., 2002; 53 37 volatile inventory for our study sites on Mars. Geologic Fagents and Thordarson, 2006]. They were first identified 54 38 features that form in the presence of water or ice are of in Viking Orbiter imagery [Allen, 1979a; Frey et al., 1979; 55 39 key interest because they provide information about the Frey and Jarosewich, 1982], and more recently from data 56 40 abundance and spatial distribution of volatiles on Mars at acquired by both the Thermal Emission Imaging System 57 41 the time of feature formation. Such features include (THEMIS) on Mars Odyssey and the high-resolution (Nar- 58 42 volcanic rootless cones, subglacial volcanoes, rampart row-Angle) Mars Orbiter Camera (MOC-NA) aboard Mars 59 43 craters, pingos, and other types of ice mounds [Carr et Global Surveyor. Rootless cones, found in abundance in 60 44 al., 1977; Allen, 1979a, 1979b; Frey et al., 1979; Iceland, form when lava flowing in an inflating pahoehoe 61 45 Lucchitta, 1981; Frey and Jarosewich, 1982; Mouginis- flow field interacts explosively with water or ice contained 62 within the substrate [Thorarinsson, 1951, 1953]. Successive 63 1Hawai`i Institute of Geophysics and Planetology, University of Hawai`i, explosive pulses eject fragments of lava and substrate from 64 Honolulu, Hawaii, USA. the explosion site to build a cratered cone of ash, scoria, 65 2 SETI Institute, Mountain View, California, USA. 66 3 spatter, and clastic substrate sediments. Once activity at a Proxemy Research, Bowie, Maryland, USA. given explosion site wanes (due to volatile depletion or a 67 cessation of lava supply), explosive interactions typically 68 Copyright 2006 by the American Geophysical Union. 0148-0227/06/2005JE002510$09.00 initiate nearby. The result is a group containing tens to 69 XXXXXX 1of16 XXXXXX BRUNO ET AL.: ROOTLESS CONES, ICE MOUNDS, AND IMPACT CRATERS XXXXXX 70 hundreds of cones [Thordarson et al., 1998; Thordarson, forces water to intrude into shallow permafrost. Upon 132 71 2000]. freezing, the water ice uplifts the overburden to form 133 72 [4] Rootless volcanic cones are distinct from primary mounds [Washburn, 1973]. Hydraulic pingos may also 134 73 scoria cones, which form over a volcanic conduit more grow as a result of hydraulic lift, where low artesian 135 74 deeply rooted in the crust to a magma source. Unlike pressure is amplified through confinement under a pingo 136 75 primary cones, rootless cones are not the source of lava [Mu¨ller, 1959; Holmes et al., 1968]. When discussing ice 137 76 flows (although they can feed rheomorphic flows that can cored mounds of uncertain origin, we use the general term 138 77 be difficult to distinguish from primary lava flows). ‘‘ice mound’’ (rather than the specific term ‘‘pingo’’) to 139 78 Figures 1a and 1b show a typical rootless cone group within include other segregated ice bodies such as palsas, hydro- 140 79 the 1783-84 Laki lava flow in Iceland. The morphology and laccoliths, icings, and ice hummocks. (See Washburn [1973] 141 80 spatial density of rootless cones provide an indication of the for a comprehensive description of these features.) We 142 81 quantity and location of water in the substrate at the time of reserve the term ‘‘pingo’’ for ice cored mounds of known 143 82 cone formation; modeling the dynamics of cone-forming hydraulic or hydrostatic origin. 144 83 explosions permits assessment of the vapor mass and [9] On Mars, potential rootless volcanic cones have been 145 84 pressure required to produce cones of the observed numbers identified in the northern lowland plains (Figure 2a), 146 85 and sizes [Greeley and Fagents, 2001]. If rootless cones can including Acidalia, Amazonis, Isidis, and Elysium Planitiae 147 86 be unequivocally identified on Mars, we will have a [Allen, 1979a; Frey and Jarosewich, 1982; Greeley and 148 87 powerful means to gauge the amount, distribution, and Fagents, 2001; Lanagan et al., 2001; Fagents et al., 2002]. 149 88 evolution of near-surface volatiles in the regolith. Possible Martian ice mounds have been identified in Gusev 150 89 [5] Similarly, the putative identification of pingos and Crater, Athabasca Valles and the Cerberus Plains (Figure 2b) 151 90 other ice mounds on Mars implies the presence of volatiles as defined by Plescia [1990], and Chryse, Acidalia, and 152 91 in the Martian regolith [Lucchitta, 1981; Parker et al., 1993; Utopia Planitiae [Lucchitta,1981;Parker et al., 1993; 153 92 Cabrol et al., 2000; Burr et al., 2005; Soare et al., 2005]. A Cabrol et al., 2000; Burr et al., 2005; Soare et al., 2005; 154 93 pingo is a type of ice cored mound, common in periglacial Page and Murray, 2006]. 155 94 environments within Alaska, Canada, and Russia. Like [10] Historically, Martian pingos have been difficult to 156 95 rootless cones, pingos can grow up to several hundreds of identify on the basis of Viking Orbiter data, in part because 157 96 meters in basal diameter and up to tens of meters in height of limited availability of high-resolution images, but also 158 97 [Washburn, 1973]. because only the most mature pingos exhibit distinctive 159 98 [6] In general, pingos form when the overburden is morphologies. Younger and/or smaller pingos are typically 160 99 domed above a segregated ice body that grows either by expressed as low-relief mounds. Only once the mound is 161 100 (1) intrusion and subsequent freezing of liquid water sufficiently upheaved does its surface begin to rupture, 162 101 injected into permafrost; (2) progressive underplating of thereby exposing the interior ice, which melts or sublimes 163 102 an ice cored mound as an underlying water reservoir to form crevices and pits at or near the summit [Washburn, 164 103 freezes; or (3) a combination of both these processes 1973; Mackay, 1979]. Moreover, these morphologies are apt 165 104 [Washburn, 1973; Mackay, 1987]. Depending on their to be confused with those of rootless cones, and the 166 105 formation mechanism, pingos are divided into two main presence of pingos or other ice mounds on Mars remains 167 106 categories: hydrostatic and hydraulic. equivocal [Theilig and Greeley,1979;Lucchitta,1981;168 107 [7] The majority of terrestrial pingos are hydrostatic Rossbacher and Judson, 1981; Squyres et al., 1992; Parker 169 108 (‘‘closed-system’’) pingos and form in response to the et al., 1993; Cabrol et al., 2000].
Details
-
File Typepdf
-
Upload Time-
-
Content LanguagesEnglish
-
Upload UserAnonymous/Not logged-in
-
File Pages16 Page
-
File Size-