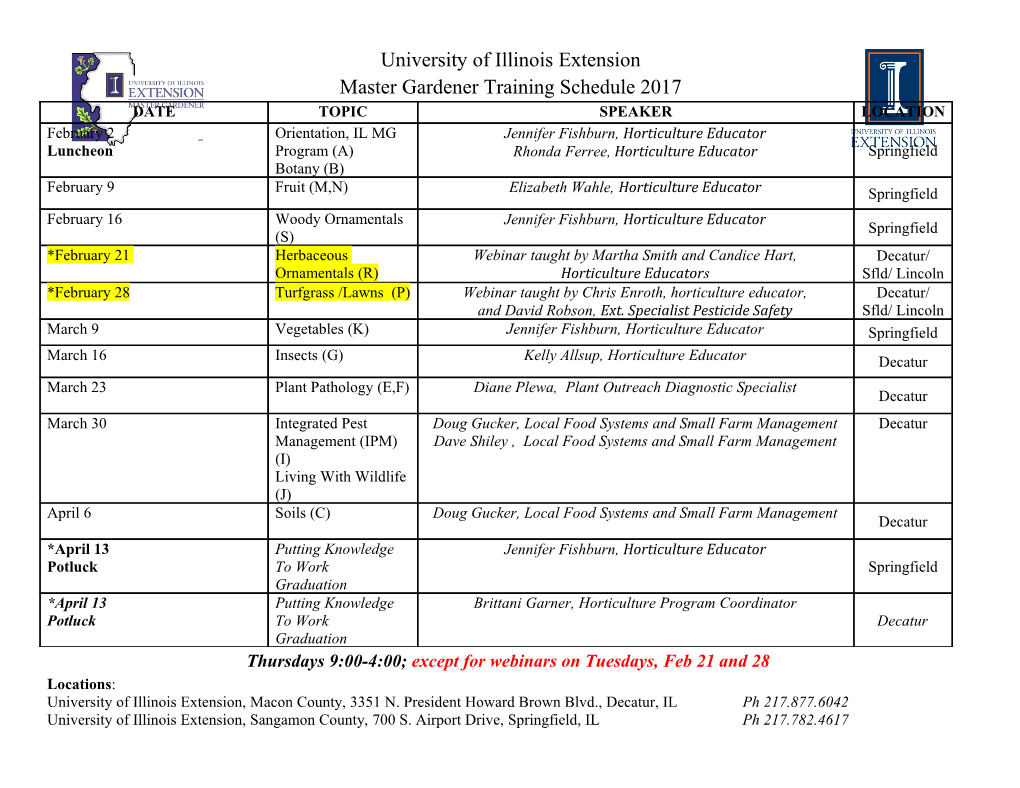
DRAFT VERSION OCTOBER 6, 2017 Typeset using LATEX twocolumn style in AASTeX61 MULTI-MESSENGER OBSERVATIONS OF A BINARY NEUTRON STAR MERGER LIGO SCIENTIFIC COLLABORATION,VIRGO COLLABORATION AND PARTNER ASTRONOMY GROUPS (Dated: October 6, 2017) ABSTRACT On August 17, 2017 a binary neutron star coalescence candidate (later designated GW170817) with merger time 12:41:04 UTC was observed through gravitational waves by the Advanced LIGO and Advanced Virgo detectors. The Fermi Gamma-ray Burst Monitor independently detected a gamma-ray burst (GRB170817A) with a time-delay of 1.7swith respect to the merger ⇠ time. From the gravitational-wave signal, the source was initially localized to a sky region of 31 deg2 at a luminosity distance +8 of 40 8 Mpc and with component masses consistent with neutron stars. The component masses were later measured to be in the range− 0.86 to 2.26 M . An extensive observing campaign was launched across the electromagnetic spectrum leading to the discovery of a bright optical transient (SSS17a, now with the IAU identification of AT2017gfo) in NGC 4993 (at 40 Mpc) less ⇠ than 11 hours after the merger by the One-Meter, Two Hemisphere (1M2H) team using the 1-m Swope Telescope. The optical transient was independently detected by multiple teams within an hour. Subsequent observations targeted the object and its environment. Early ultraviolet observations revealed a blue transient that faded within 48 hours. Optical and infrared observations showed a redward evolution over 10 days. Following early non-detections, X-ray and radio emission were discovered at the ⇠ transient’s position 9 and 16 days, respectively, after the merger. Both the X-ray and radio emission likely arise from a ⇠ ⇠ physical process that is distinct from the one that generates the UV/optical/near-infrared emission. No ultra-high-energy gamma rays and no neutrino candidates consistent with the source were found in follow-up searches. These observations support the hypothesis that GW170817 was produced by the merger of two neutron stars in NGC 4993 followed by a short gamma-ray burst (GRB170817A) and a kilonova/macronova powered by the radioactive decay of r-process nuclei synthesized in the ejecta. 2 1. INTRODUCTION holes. These hints included: (i) their association with both Over 80 years ago Baade & Zwicky (1934) proposed the elliptical and star forming galaxies (Barthelmy et al. 2005; idea of neutron stars, and soon after, Oppenheimer & Volkoff Prochaska et al. 2006; Berger et al. 2007; Ofek et al. 2007; (1939) carried out the first calculations of neutron-star mod- Troja et al. 2008; D’Avanzo et al. 2009; Fong et al. 2013), els. Neutron stars entered the realm of observational astron- due to a very wide range of delay times, as predicted the- omy in the 1960s, by providing a physical interpretation of oretically (Bagot et al. 1998; Fryer et al. 1999; Belczyn- X-ray emission from Scorpius X-1 (Giacconi et al. 1962; ski et al. 2002); (ii) a broad distribution of spatial offsets Shklovsky 1967) and of radio pulsars (Hewish et al. 1968; from host-galaxy centers (Berger 2010; Fong & Berger 2013; Gold 1968, 1969). Tunnicliffe et al. 2014),which was predicted to arise from The discovery of a radio pulsar in a double neutron-star supernova kicks (Narayan et al. 1992; Bloom et al. 1999); system by Hulse & Taylor (1975) led to a renewed interest in and (iii) the absence of associated supernovae (Fox et al. binary stars and compact-object astrophysics, including the 2005b; Hjorth et al. 2005b,c; Soderberg et al. 2006; Ko- development of a scenario for the formation of double neu- cevski et al. 2010; Berger et al. 2013a). Despite these strong tron stars and the first population studies (Flannery & van hints, proof that sGRBs were powered by neutron-star merg- den Heuvel 1975; Massevitch et al. 1976; Clark 1979; Clark ers remained elusive, and interest intensified in following up et al. 1979; Lipunov et al. 1987; Dewey & Cordes 1987; gravitational-wave detections electromagnetically (Metzger for reviews see Kalogera et al. 2007; Postnov & Yungelson & Berger 2012; Nissanke et al. 2013). 2014). The Hulse-Taylor pulsar provided the first firm evi- Evidence of beaming in some sGRBs was initially found dence (Taylor & Weisberg 1982) for the existence of gravi- by Soderberg et al. (2006); Burrows et al. (2006) and con- tational waves (Einstein 1916, 1918), and sparked a renais- firmed by subsequent sGRB discoveries (see compilation and sance of observational tests of general relativity (Damour & analysis by Fong et al. 2015 and also Troja et al. 2016). Taylor 1991; Taylor et al. 1992; Damour & Taylor 1992; Wex Neutron-star binary mergers are also expected, however, to 2014). Merging binary neutron stars were quickly recognized produce isotropic electromagnetic signals, which include: to be promising sources of detectable gravitational waves, (i) early optical and infrared emission, a so-called kilo- making them a primary target for ground-based interferomet- nova/macronova (hereafter kilonova) (Li & Paczynski´ 1998; ric detectors (see Abadie et al. 2010, for an overview). This Kulkarni 2005; Rosswog 2005; Metzger et al. 2010; Roberts motivated the development of accurate models for the two- et al. 2011; Kasen et al. 2013; Barnes & Kasen 2013; Tanaka body, general-relativistic dynamics (Blanchet et al. 1995; & Hotokezaka 2013; Grossman et al. 2014; Barnes et al. Buonanno & Damour 1999; Pretorius 2005; Campanelli et al. 2016; Tanaka 2016; Metzger 2017) due to radioactive de- 2006; Baker et al. 2006; Blanchet 2014) that are critical for cay of rapid neutron-capture process (r-process) nuclei (Lat- detecting and interpreting gravitational waves (Abbott et al. timer & Schramm 1974, 1976) synthesized in dynamical and 2016a,b, 2017a,b,c). accretion-disk-wind ejecta during the merger; and (ii) de- In the mid-1960s gamma-ray bursts (GRBs) were discov- layed radio emission from the interaction of the merger ejecta ered by the Vela satellites, and their cosmic origin was first with the ambient medium (Nakar & Piran 2011; Piran et al. established by Klebesadel et al. (1973). GRBs are classi- 2013; Hotokezaka & Piran 2015; Hotokezaka et al. 2016). fied as long or short, based on their duration and spectral The late-time infrared excess associated with GRB130603B hardness (Dezalay et al. 1992; Kouveliotou et al. 1993). Un- was interpreted as the signature of r-process nucleosynthesis covering the progenitors of GRBs has been one of the key (Berger et al. 2013b; Tanvir et al. 2013), and more candidates challenges in high-energy astrophysics ever since (Lee & were identified later (for a compilation see Jin et al. 2016). 1 Ramirez-Ruiz 2007). It has long been suggested that short Here we report on the global effort that led to the first joint GRBs might be related to neutron-star mergers (Paczynski detection of gravitational and electromagnetic radiation from a single source. A 100 s-long gravitational-wave signal 1986; Goodman 1986; Eichler et al. 1989; Narayan et al. ⇠ 1992). (GW170817) was followed by a sGRB (GRB170817A) and In 2005 the field of short gamma-ray burst (sGRB) stud- an optical transient (SSS17a/AT2017gfo) found in the host ies experienced a breakthrough (for reviews see Nakar 2007; galaxy NGC 4993. The source was detected across the elec- Berger 2014) with the identification of the first host galax- tromagnetic spectrum – in the X-ray, ultraviolet, optical, in- ies of sGRBs and multi-wavelength observation (from X- frared, and radio bands – over hours, days, and weeks. These ray to optical and radio) of their afterglows (Gehrels et al. 2005; Fox et al. 2005a; Villasenor et al. 2005; Hjorth et al. 1 This effort was established in the time between initial and advanced 2005a; Berger et al. 2005). These observations provided LIGO-Virgo observations. Partners have followed up binary-black-hole de- strong hints that sGRBs might be associated with merg- tections, starting with GW150914 Abbott et al. 2016, but have discovered no firm electro-magnetic counterparts to those events. ers of neutron stars with other neutron stars or with black MMA — LIGO-P1700294-V43 observations support the hypothesis that GW170817 was around right ascension ↵(J2000.0) = 12h57m and declina- produced by the merger of two neutron stars in NGC4993, tion δ(J2000.0) = 17◦510. Soon after, a coherent anal- − followed by a sGRB and a kilonova powered by the radioac- ysis (Veitch et al. 2015) of the data from the detector net- tive decay of r-process nuclei synthesized in the ejecta. work produced a sky map that was distributed at 23:54:40 UTC (The LIGO Scientific Collaboration et al. 2017c), con- 2. A MULTI-MESSENGER TRANSIENT sistent with the initial one: a 34 deg2 sky region at ' On August 17, 2017 12:41:06 UTC the Fermi Gamma- 90% credibility centered around ↵(J2000.0) = 13h09m and ray Burst Monitor (GBM) (Meegan et al. 2009) on-board δ(J2000.0) = 25◦370 − flight software triggered on, classified, and localized, a The offline gravitational-wave analysis of the LIGO- GRB. A Gamma-ray Coordinates Network (GCN) No- Hanford and LIGO-Livingston data identified GW170817 tice (Fermi-GBM 2017) was issued at 12:41:20 UTC an- with a false-alarm-rate of less than one per 8.0 104 years (Ab- ⇥ nouncing the detection of the GRB, which was later des- bott et al. 2017c). This analysis uses post-Newtonian wave- ignated GRB170817A (von Kienlin et al. 2017). Approxi- form models (Blanchet et al. 1995, 2004, 2006; Bohe´ et al. mately 6 minutes later, a gravitational-wave candidate (later 2013) to construct a matched-filter search (Sathyaprakash designated GW170817) was registered in low latency (Can- & Dhurandhar 1991; Cutler et al. 1993; Allen et al. 2012) non et al. 2012; Messick et al.
Details
-
File Typepdf
-
Upload Time-
-
Content LanguagesEnglish
-
Upload UserAnonymous/Not logged-in
-
File Pages71 Page
-
File Size-