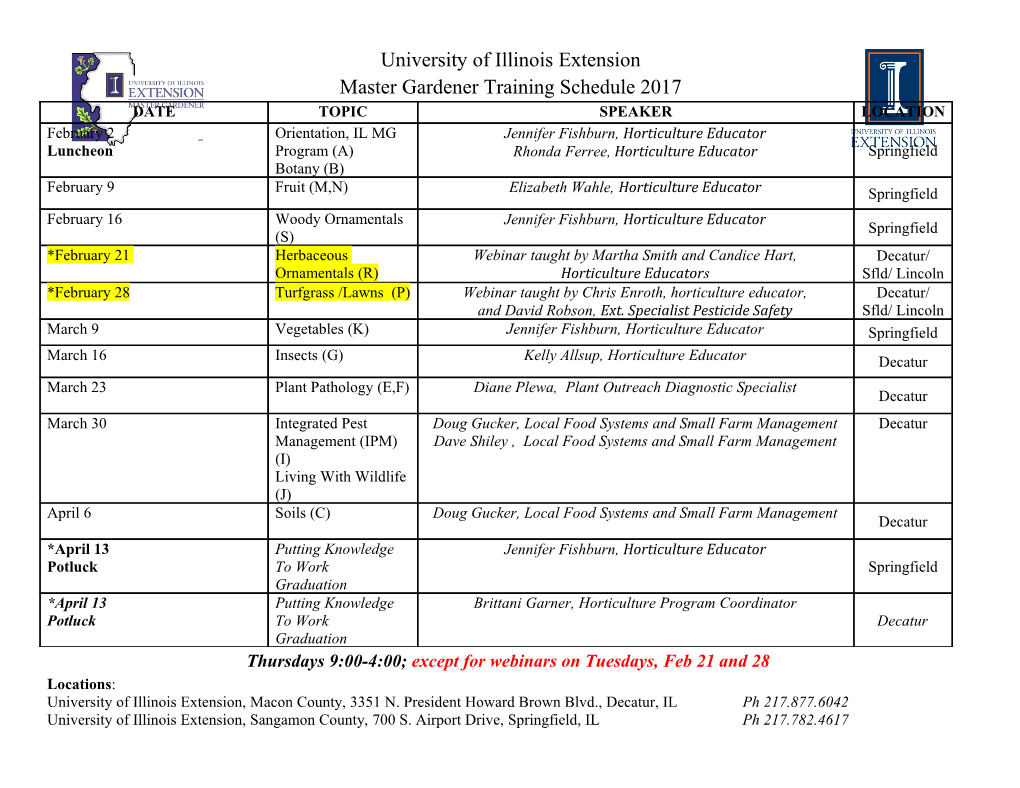
Liquid Scintillation Counting As unstable nuclides spontaneously change into more stable species, they emit charged particles and/or electromagnetic radiation (Alpha particles, Beta particles (electrons), Gamma rays or X-rays). Gamma rays and X-rays are identical - the gamma ray originating from the nucleus and the X-ray from the orbital electrons. Nuclides that emit gamma or X-ray are best counted with solid crystal scintillator such as sodium iodide. Beta particles are best counted by liquid scintillation (LS) methods in which the nuclide is intimately mixed with a scintillator. Nuclides used in radioimmunoassays of clinical interest that emit gamma or X-rays include 125I, 131I, 51Cr, 57Co. Some radionuclides that emit gamma or X- ray also emit beta particles or electrons during their decay (131I, 60Co, 53Fe). The total number of atoms of the radionuclide present which spontaneously decay in a unit time is the absolute activity of that sample, usually expressed as disintegration per minute DPM. Because of some factors in the environment, every disintegration is not detected by the photo-multiplier tube. Since the counts per minute (CPM) are always a fraction of the actual disintegration per minute, the efficiency of the counting system is expressed as - % Efficiency = CPM / DPM x 100 1 Procedure for the Use of Liquid Scintillation Counters: • The radionuclide emits a beta particle which upon collision, transfers its energy to an aromatic organic solvent molecule; • The π -electron cloud of these solvent molecules accept this energy and becomes excited to a higher energy level; • Next, the energy from the activated solvent molecule is transferred to another organic molecule serving as the scintillator (Solute); • The scintillator, upon return to ground state, emits light, which is detected using appropriate PMT. Processes in Liquid Scintillation Counting Excited states Solvent Ionization Solvent Diffusion Re-combination & Migration Internal Conversions Solute π σ Photon Emission Radioactive Quenching Sample β Emitter 2 LSC Scintillator Guide Fluorescence Scintillator Acronym emission function maximum, nm BBOT Prim &Sec. 425-435 Butyl-PBD Primary 360-365 PBD Primary 360-370 PPO Primary 360-365 p-TP Primary Bis-MSB Secondary 420-430 dimethyl Secondary 425-430 POPOP Solvent PPO* POPOP* Touline PMT Sensitivity Light Emission Wavelength 450 nm The more energetic the beta, more collisions occur, thus, yielding the greater number, N, of excited solvent molecules. Since the emitted ultraviolet radiation is not suitable for detection in the 200-260 nm wavelength range, fluorescent organic materials are added to the solvent which are capable of absorbing the UV radiation. These fluors re-emit the energy at longer wavelengths. The fluor absorbs the short wavelength energy and re-emits it at a longer wavelength, preferably in the visible region. 3 Detection Liquid Scintillation counter: A comparison of detection limits of some other methods of analysis with those detection limits obtained by using radioisotopes are shown below. Common methods of analysis METHOD OF ANALYSIS LIMIT OF DETECTABILITY REMARKS IR Spectroscopy 1015 molecules Non-destructive UV Spectroscopy 1015 molecules Non-destructive Atomic Absorption 1013 atoms Destructive Flame Emission 1013 atoms Destructive Gas Chromatography 1013 atoms Destructive Radio-Isotopes Non-destructive 14C 1011 atoms (half life 5730 yr.) 3H 109 atoms (half life 12.26 yr.) 32P 6 x 106 atoms (half life 14.29 d) Characterization of Beta Energy Spectrum- Distribution of beta particles emitted from a single type of nucleus Ep dN H# 1. Maximum Particle Energy (Emax) dE E max 2. Pulse Height at Peak Energy (Ep) 3. Inflection Point (H# number) Energy (keV) 4. Average Pulse Height – Spectral Index of the Sample (SIS) n(x) u Σ x=0 x * n(x) SIS = K Σu x x=0 n(x) Energy (keV) 4 Commonly used beta emitting isotopes ISOTOPE EMAX (MEV) 3H 0.018 14C 0.156 35S 0.168 45Ca 0.250 32P 1.710 131I 0.610 (F/87% BETA ACTIVITY) Tritium beta particle detection requires – • A more sensitive detector • High efficiency energy converter If a beta emitting material is interspersed with an aromatic solvent, such as benzene, toluene, or P-xylene, the emitted particle produces excitation and ionization in the solvent. Electrons in the conjugated carbon-carbon double bonds of these aromatic solvent molecules are excited to a high energy state by absorbing some of the beta particles energy and upon returning to the lower energy ground state, release their energy in the form of light. This conversion of kinetic energy to light is referred to as the scintillation process Beta Collision Process Excited Excited Solute Solvent Emitted Photons Ground Ground Quenching Level Level Solvent Solute Sample 5 Solvents Solvents and fluors used in liquid scintillation counting Solvent Fluors Dioxane PPO Toluene dimethyl - popopbutyl PBO p-Xylene PBBO. Some key properties are: a) Purity b) High solubility for sample and the fluors, c) High transfer efficiency for conversion of particles energy into excited molecules d) Chemical stability. Since small amounts of certain substances can produce a dramatic loss of scintillation efficiency, highly purified solvents are necessary. Toluene P PPO Emission h 1. PPO Absorption o Emission t o n I n t e n s i t y 0 480 280 Wavelength (nm) 6 The Excitation Process A. Photon Emission 1. The primary excitation in liquid scintillation counting is produced by a charged particle (beta) passing through or stopped by the scintillation solution. This transfer of energy results in the production of a large number of excited molecules and ion pairs. The ions recombine with electrons to produce additional excited molecules. 2. About 60% of the fluorescing molecules are generated by this mechanism. 3. The remaining 40% are the result of direct excitation of the solvent molecule. 4. The amount of light produced by the solution (scintillation yield) will depend on the concentration of the scintillator (fluor). Excimers are the dimers formed by a pair of excited molecule and an unexcited molecule of the same kind. In this equilibrium high concentration and low temperatures favor the excimer formation. The excimer has lower energy than the monomer so that it’s fluorescence light has the longer wavelength. B. Quenching Effect Quench - anything that interferes with the scintillation process in any of the above four steps prevents light from reaching the PMT, results in a loss in the number of recorded counts and in the apparent energy. The Causes of Quench are- 1) Impurities in the cocktail material, 2) Insufficient amounts of solute or solvent 3) Too much sample volume. Color quenching occurs when colored substances, present in the sample, absorb the light emitted by the scintillator (fluors) before it can escape the vial and reach PMT. The net effect of Color quenching is the reduction in CPM registered by the counter. 7 Scintillation yield The scintillation yield is defined as the total energy emitted by the solution (as light or photons) divided by the energy of the particle that excites the solution to fluoresce. Scintillation efficiency Sx is defined as Sx = Nph . Eph/ Eex Where Nph = average number of photons Eph = average energy of photons Eex = energy of the exciting particle The solvent determines the overall scintillation efficiency. Efficiency has different value for • each solvent • different isotopes in the same solvent From the scintillation efficiency - it is possible to calculate the average number of photons (Nph ) produced by a particle of energy Eex. Nph = Sx Eex / Eph The average energy of the photons Eph can be calculated from the average wavelength of fluorescence. For the scintillator, PPO, the average wavelength of fluorescence is 3900 oA. Eph = hν = h C/λ 5 10 5 Eph = (4.143 x 10 eV) (2.99 x 10 )/(3.8 x 10- ) At about 7 gm/liter of PPO in toluene, the transfer efficiency is about 100% and the quantum efficiency is 1.0 3 Nph = (0.04) (10 eV/KeV) (Eex)/(3.2 eV) Nph = 12.5 KeV (Eex) The number of photoelectrons produced at the photo-cathode is now given by: No. of photoelectrons = Nph x photo-cathode efficiency The photo cathode efficiency is 28% for the bralkalu type phototube currently in use. 8 Quench Indicating Parameters 1. Based on Sample Spectrum: A parameter is derived from the sample spectrum. Quench correction curve is generated by measuring this parameter on a set of standard sources with different quenching effects. The unknown sample measurement is corrected for the quenching using this curve. Different parameters are - • Spectral Index Sample (SIS): Average Energy of sample spectrum. • Sample channel Ratio (SCR) Ratio of counts in two channels selected in such a way that the ratio of counts indicates the quenching effect. Channel A CPM Channel B Energy • Spectral Quench Parameter of Isotope (SQP): The spectrum is transformed by log E operation. The Sample Index Spectrum of this spectrum defines the SQP and is the mean log energy of the spectrum. SQP – Average Counts / log energy Minute Log (Energy) 9 B. Quenching Effects 1. Self-quenching: Increasing the concentration beyond the optimal level will usually result in an appreciable reduction in scintillation yield. 2. Solute (Scintillator)-quenching: The reduction in efficiency with increasing scintillator concentration appears to depend on the ability of the scintillator to achieve a co-planer configuration. This may result in either an increase or decrease in measured scintillation efficiency depending on the form (monomer or dimer) that is more favorable. Inherent interference in Liquid Scintillation Spectrum Analyses 1. Quenching – Two main types are - Chemical: causes energy loss in transfer from Solvent to solute. Colour : Causes Attenuation of photons. UnUnquenchedquenched CPMU & SISU of quenched spectrum of a sample are used to Quenched obtain the DPMU by reference to a n(x) graph between the Efficiency and SIS.
Details
-
File Typepdf
-
Upload Time-
-
Content LanguagesEnglish
-
Upload UserAnonymous/Not logged-in
-
File Pages48 Page
-
File Size-