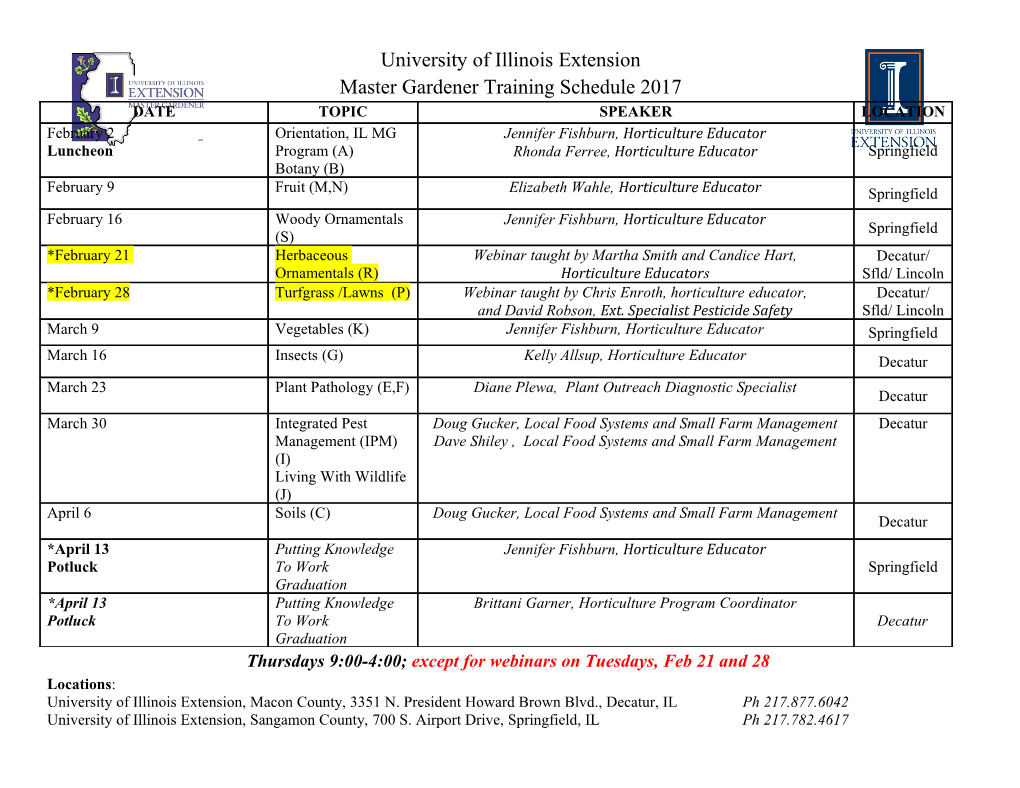
Synthesis of FMN Analogues as Probes for the Photocycle of Avena sativa Andrew Wood Submitted in fulfilment of the requirement for the degree of Doctor of Philosophy School of Chemistry, Cardiff University February, 2014 Declaration This work has not been submitted in substance for any other degree or award at this or any other university or place of learning, nor is being submitted concurrently in candidature for any degree or other award. Signed ………………………………………… (candidate) Date ………………………… STATEMENT 1 This thesis is being submitted in partial fulfilment of the requirements for the degree of PhD. Signed ………………………………………… (candidate) Date ………………………… STATEMENT 2 This thesis is the result of my own independent work/investigation, except where otherwise stated (see below). Other sources are acknowledged by explicit references. The views expressed are my own. Signed ………………………………………… (candidate) Date ………………………… STATEMENT 3 I hereby give consent for my thesis, if accepted, to be available for photocopying and for inter- library loan, and for the title and summary to be made available to outside organisations. Signed ………………………………………… (candidate) Date ………………………… i Declaration of Contributions I confirm that the work presented within this thesis was performed by the author, with exceptions summarised here, and explicitly referenced in the relevant sections of the thesis. The original construction of several plasmid vectors used for the expression of recombinant proteins was not performed, as they were freely available (from previous work), and donated to me. Their original preparation is summarised below. Furthermore, during a short side- project the dephosphorylation of 5-deazaFAD was performed (on an analytical scale) by a collaborator (Dr. Hannah Collins, University of Kent) using commercially available Phosphodiesterase I from Crotalus atrox. This is described in section 7.1.5 in order to support other results independently obtained during this research, and full reference is made to the contributing experimenter. C. ammoniagenes RfK-FADS (pMAL-C2-RibF-Ca) The plasmid vector pMAL-C2 containing the gene for Ca-RfK-FADS was originally prepared by others including the project supervisor (Gerald Richter, University of Cardiff), and its sequence and details are reported in a freely available journal1. S. pombe RfK (pET14b-SpRfK): The original cloning of the S. pombe riboflavin kinase from the commercially available gene (GenBank®2,3 reference: NM_001023386.2) into the plasmid expression vector pET-14b was performed by Dr. Hannah Collins (University of Kent), and the construct was gifted to me during a short side-project. A.sativa PHOT1-LOV2 (pNCO113-HISACT(C49S)-AsLOV2) The creation of the plasmid pNCO113-HISACT(C49S)-AsLOV2(WT) was performed by others including the project supervisor (Gerald Richter); this is described in freely available literature1,4, and its sequence added to the GenBank®2,3 (reference: ef493211.1). This construct allowed expression of the wild-type AsLOV2 domain as a hisactophilin fusion protein, and was also used as the basis for subsequent mutagenesis of the AsLOV2 domain. ii Abstract The effect of light on plants is fundamental to our understanding of the biological world. Photo-activated processes within botanical cells are controlled by two distinct colours; red light provides the energy required for photosynthesis, and blue light stimulates responses of the cell (growth, stomatal opening, etc.) necessary for biological progression. The key step in blue-light controlled processes has recently been determined to be the formation of a covalent bond between a tightly-bound molecule of flavin and a conserved cysteine residue within a photosensitive protein domain, which causes a physical change to the protein structure upon irradiation. This research was undertaken to examine light-stimulated covalent bond formation in phototropic proteins using two approaches. Firstly, two analogues of riboflavin (5-deazariboflavin and 1-deazariboflavin) were chemically synthesised, converted to the appropriate cofactor form (flavin mononucleotide; FMN), and incorporated into the photosensitive domain. The results of this were startling; while 1-deazaFMN inactivated the photosensitive domain to all wavelengths of light, 5-deazaFMN allowed control of the domain’s behaviour using two separate wavelengths of light, creating a biophotonic nanoswitch. The second approach was based upon mutagenesis of the amino acid responsible for bond formation, introducing several alternative residues in place of the reactive cysteine. All mutants were found to be inactive to irradiation (including those incorporating either of the deazaFMN analogues), providing further evidence to support the current “radical pair” mechanism of photoadduct formation. During the synthesis of the flavin analogues, several reactions were found to be inefficient, and a large number of alternative reactions were studied. Additionally, a new route to several flavin analogues was devised and tested, and while unsuccessful, provides a foundation upon which to base a future examination of their synthesis. Finally, the synthesis of a novel riboflavin analogue (5-deaza-8-demethyl-riboflavin) was completed, allowing comparison with the synthesis of 5-deazariboflavin. iii Acknowledgements Firstly, I wish to thank my supervisor, Professor Gerald Richter, for his introduction to flavin chemistry. His knowledge in this field and his confidence in my abilities was fundamental in my decision to attempt the research presented in this thesis. I would like to thank Dr. James Redman and Professor Rudolf Allemann for allowing me space to work within their laboratories, and for their thought-provoking questioning throughout my research. I would like to thank Professor Nick Tomkinson (University of Strathclyde), and Professor Mark Bagley (University of Sussex) for mentoring me during their readerships in Cardiff, as well as the technical and administrative staff (past and present) in the School of Chemistry for their support. In particular I would like to thank Dr. Robert Jenkins and Mr. Robin Hicks for their assistance with all aspects of NMR Spectroscopy and Mass Spectrometry. I wish to thank Dr. Robert Mart, and a fellow PhD student whom I worked alongside, Dr. Steven Hill, for their assistance in the development of ideas and their numerous suggestions. Their support led to a vast improvement in my knowledge of chemical synthesis, and greatly improved the scope of this work. I also wish to extend thanks to all members of the Chemical Biology group in the School of Chemistry for their immeasurable help with the biological elements of this work. I began this project with no prior practical experience of this field, and have benefitted greatly by their patient instruction in the techniques and methods used for the molecular biology performed during my research. Furthermore, I would like to thank my friends (Seni Chanapai, William Dawson, Sarah Adams, Daniel Grundy and Dilruba Meah) for their assistance and reassurance, and my partner Sally Pemberton, for her continued encouragement. Finally, I must give thanks to my parents and friends for their love, encouragement and support throughout the course of my education, which is truly appreciated. Their contributions have been fundamental in my development both as a researcher and as an individual, and without which it would have been impossible to complete this project. iv List of Abbreviations In addition to abbreviations in common occurrence throughout current literature, the following abbreviations are in use throughout this thesis. These are divided for convenience into abbreviations used for chemicals, and for techniques and methods. Chemical Abbreviations 18-C-6 18-crown-6 4-DMAP 4-dimethylaminopyridine, also known as N,N- dimethylaminopyridine Ac acetyl; functional group AcCl acetyl chloride Ac2O acetic anhydride AcOH acetic acid ADP adenosine-5’-diphosphate AMP adenosine-5’-monophosphate APS ammonium persulphate Arg arginine (R); amino acid Ar aryl; functional group Asp aspartic acid (D); amino acid ATP adenosine-5’-triphosphate Bn benzyl; functional group Boc tert-butyloxycarbonyl; functional / protecting group Boc anhydride di-tert-butyl dicarbonate CAM cerium ammonium molybdate CAN cerium ammonium nitrate Cys cysteine (C); amino acid CTP cytidine-5’-triphosphate DCM dichloromethane dioxane 1,4-dioxane DABCO diazabicyclo[2.2.2]octane dATP deoxyadenosine triphosphate, see dNTP v dCTP deoxycytidine triphosphate, see dNTP dGTP deoxyguanosine triphosphate, see dNTP DIPEA N,N-diisopropylethylamine DMAP N,N-dimethylaminopyridine DMF N,N-dimethylformamide DMPU 1,3-dimethyl-3,4,5,6-tetrahydro-2(1H)-pyrimidinone DMSO dimethylsulphoxide DNA deoxyribonucleic acid DNPH 2,4-dinitrophenylhydrazine dNTP deoxynucleotide triphosphate (ATP, GTP, CTP, TTP) DTT dithiothreitol dTTP deoxythymidine triphosphate, see dNTPs EtBr* ethidium Bromide (not bromoethane; by convention) EDTA ethylenediaminetetraacetic acid Et2O diethyl ether EtOAc ethyl acetate EtOH ethanol FAD flavin adenine dinucleotide FMN flavin mononucleotide GTP guanosine-5’-triphosphate GuHCl guanidinium hydrochloride GuSCN guanidinium thiocyanate HATU 1-[Bis(dimethylamino)methylene]-1H-1,2,3-triazolo[4,5- b]pyridinium 3-oxid hexafluorophosphate HCl hydrochloric acid HEPES 4-(2-hydroxyethyl)-1-piperazineethanesulfonic acid IPTG isopropyl-1-thio-β-D-galactopyranoside KOH potassium hydroxide LB (growth media) Leuria-Bertani media for bacterial growth Me methyl; functional group MeOH methanol
Details
-
File Typepdf
-
Upload Time-
-
Content LanguagesEnglish
-
Upload UserAnonymous/Not logged-in
-
File Pages269 Page
-
File Size-