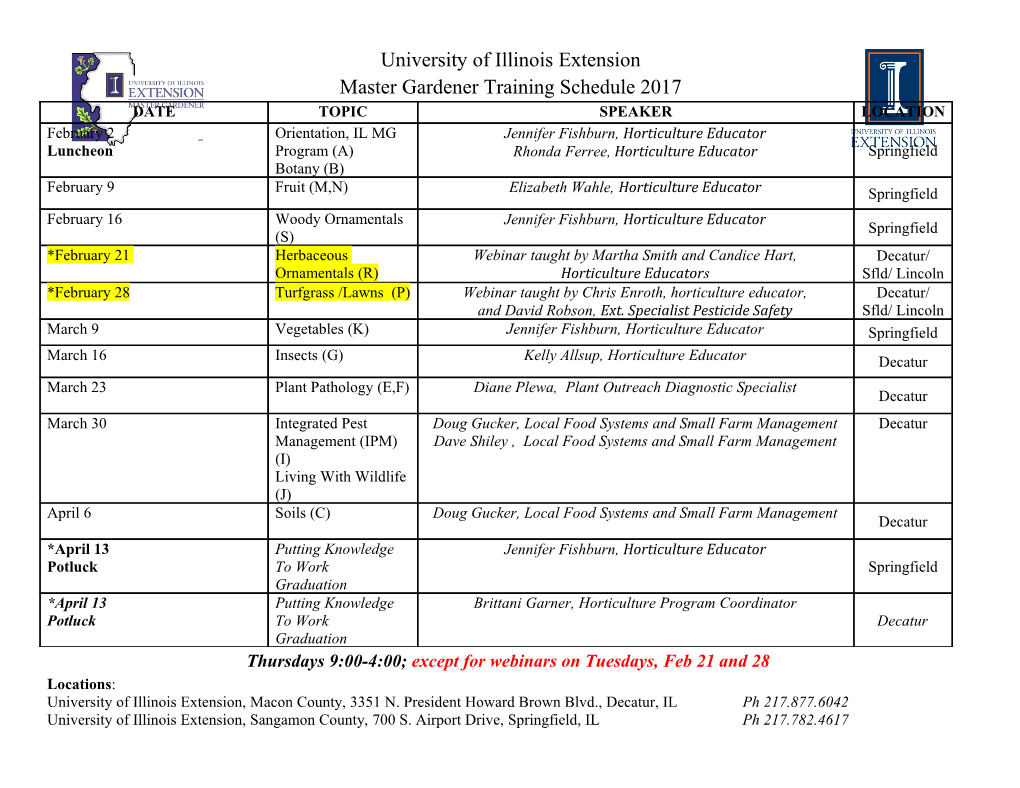
Neuropharmacology 44 (2003) 1–7 www.elsevier.com/locate/neuropharm Review Pharmacology of neuronal background potassium channels Florian Lesage Institut de Pharmacologie Mole´culaire et Cellulaire, CNRS UMR6097, 660, route des lucioles, Sophia Antipolis, 06560 Valbonne, France Received 10 May 2002; received in revised form 9 August 2002; accepted 26 September 2002 Abstract Background or leak conductances are a major determinant of membrane resting potential and input resistance, two key components of neuronal excitability. The primary structure of the background K+ channels has been elucidated. They form a family of channels that are molecularly and functionally divergent from the voltage-gated K+ channels and inward rectifier K+ channels. In the nervous system, the main representatives of this family are the TASK and TREK channels. They are relatively insensitive to the broad- spectrum K+ channel blockers tetraethylammonium (TEA), 4-aminopyridine (4-AP), Cs+, and Ba2+. They display very little time- or voltage-dependence. Open at rest, they are involved in the maintenance of the resting membrane potential in somatic motoneu- rones, brainstem respiratory and chemoreceptor neurones , and cerebellar granule cells. TASK and TREK channels are also the targets of many physiological stimuli, including intracellular and extracellular pH and temperature variations, hypoxia, bioactive lipids, and neurotransmitter modulation. Integration of these different signals has major effects on neuronal excitability. Activation of some of these channels by volatile anaesthetics and by other neuroprotective agents, such as riluzole and unsaturated fatty acids, illustrates how the neuronal background K+ conductances are attractive targets for the development of new drugs. 2002 Elsevier Science Ltd. All rights reserved. Keywords: Leak channels; Resting potential; Excitability Contents 1. Introduction . 1 2. The two-pore- domain K+ channels . 2 3. TASK channels in the nervous system . 3 4. TREK channels in the nervous system . 4 5. Modulation of neuronal background K+ channels by clinically relevant compounds . 5 6. Conclusion . 6 1. Introduction currents involved in action potential generation, these authors proposed a voltage-insensitive leak current as the The existence of background conductances in neu- basis of the resting membrane potential. Subsequently, rones was originally postulated by Hodgkin and Huxley it was shown that the resting potential in different types (1952). In addition to the voltage-sensitive Na+ and K+ of neurones depended primarily on K+-selective currents showing a relative insensitivity to classical K+ channel blockers (Baker et al., 1987; Jones, 1989; Premkumar et E-mail address: [email protected] (F. Lesage). al., 1990; Shen et al., 1992; Koh et al., 1992; Koyano 0028-3908/03/$ - see front matter 2002 Elsevier Science Ltd. All rights reserved. doi:10.1016/S0028-3908(02)00339-8 2 F. Lesage / Neuropharmacology 44 (2003) 1–7 et al., 1992; Theander et al., 1996). For example, in mye- domains are crucial for the formation of the pore selec- linated nerve, different K+ conductances can be success- tivity filter. Given that K+ subunits with one P domain ively removed by sequential applications of TEA, 4-AP are active as tetramers and that four P domains form the and Cs+; but treated axons still exhibit strong outward K+-selectivity filter (Doyle et al., 1998), it was hypoth- rectification suggesting that residual K+ conductance is esised early that leak K+ channels with two P domains present. The conductance, which is believed to set the were active as dimers. As expected, TWIK1 does form resting potential, is voltage-independent but outwardly dimers (Lesage et al., 1996b). These multimers contain rectifying, as expected from constant field theory (Baker an interchain disulfide bridge. The cysteine residue et al., 1987). This type of current is easily distinguish- involved in this bond is part of the extracellular loop able from the voltage-sensitive inwardly rectifying K+ located between the first membrane-spanning segment currents that play a similar role in cardiac and skeletal (M1) and the first P domain (P1). The predicted structure muscle cells (Hille, 1992). Until recently, neuronal back- of this M1P1 loop is an alpha-helix containing a regular ground currents received only a fraction of the attention occurrence of hydrophobic and charged residues. This that was devoted to the voltage-gated and Ca2+- sensitive profile is typical of interdigitating helices that interact K+ currents, but the recent cloning of a new family of through hydrophobic interactions. The regular occur- K+ channels has permitted a detailed characterisation of rence of hydrophobic and charged residues is conserved the electrophysiological and pharmacological properties, in the M1P1 loops of all the TWIK-related subunits. The and regulation of these currents (for reviews see Lesage cysteine residue and the ability to form covalent disul- and Lazdunski, 2000; Patel and Honore´, 2001). Electro- fide-dridged dimers are also conserved in the majority physiology of the corresponding conductances in vivo, of these subunits (Lesage et al., 2001). A functional in association with in situ hybridization and immunohis- approach has recently demonstrated that TASK1 tochemistry, has revealed a broad distribution of these (although it lacks this cysteine) is also active as a dimer channels in the nervous system. Another major result is (Lopes et al., 2001). In addition, the covalent dimeriz- the tight and specific regulation of these channels by a ation of some of these channels has been confirmed by variety of physical and chemical stimuli, suggesting that Western blot analysis of native proteins (Lesage and precise tuning of their activity is associated with cell- Lazdunski, 2000; Reyes et al., 2000; Hervieu et al., specific regulation of neuronal activity. 2001). These dimers contain four P domains, two P1 and two P2, supporting the idea that both P domains are functional and are involved in the formation of the ionic 2. The two-pore- domain K+ channels pore. In the leak channels, the first P1 domain can accommodate residues that are never observed in the K+ channels form the largest family of ion channels. one-P channels and that can suppress the channel activity More than 70 genes encoding pore-forming subunits when introduced in these channels. The unusual sym- have been identified in the human genome. These sub- metry, resulting from dimerization, probably provides an units are organised into three main families according evolutionary flexibility that is not possible with the to their predicted membrane topology. The two largest tetrameric symmetry of one-P-domain channels. Two-P- families comprise subunits with six or two membrane- domain channels are mainly active as homodimers but spanning segments and one pore (P) domain (Jan and a recent study suggest that TASK1 and TASK3, in parti- Jan, 1997). These subunits assemble as homo- or heterot- cular, are able to form heterodimers (Czirjak and etramers to form active channels belonging to different Enyedi, 2002). functional groups including the extensively characterised Two-P-domain K+ channels are present in all exam- voltage-gated K+ channels, Ca2+-dependent K+ channels, ined tissues. However, each channel has its own profile ATP-sensitive K+ channels, G-protein-coupled K+ chan- of expression giving to each tissue a unique channel nels, and inward rectifiers. The third family of pore-for- combination (Lesage and Lazdunski, 2000; Medhurst et ming subunits was discovered by DNA database mining. al., 2001). In human brain, the most represented two- The first channel to be cloned was TWIK1 (Lesage et al., P-domain K+ channels are TWIK1, KCNK7, TASK1, 1996a), subsequently followed by 13 additional TWIK- TASK3, TREK1, TREK2 and TRAAK (Fig. 1) related channels in human (Fig. 1). The corresponding (Medhurst et al., 2001). TWIK1, KCNK7, TASK3 and genes, designated KCNK1 to 17, are only distantly TRAAK are predominantly expressed in the CNS, related to the other K+ channel genes in evolution whereas TASK1 and TREK2 are equally present in the (Lesage et al., 1996a; Patel and Honore´, 2001; Girard et CNS and peripheral tissues (Medhurst et al., 2001). In al., 2001; Karschin et al., 2001). the brain, each channel displays a unique pattern of The TWIK1-related proteins are 300–500 residues expression with some striking differences between spec- long and share similar hydropathic profiles predicting ies. For example, TASK3, which is nearly exclusively four membrane-spanning segments. The most salient expressed in the cerebellum in human (Medhurst et al., feature is the presence of two P domains per subunit. P 2001), is found more widely in rodent brain with high F. Lesage / Neuropharmacology 44 (2003) 1–7 3 Fig. 1. The two-P-domain K+ channels form different structural and functional subclasses. The dendrogram has been produced by Treeview using a ClustalW alignment of human sequences. levels of expression in cerebellar granule neurones, play only little time- or voltage-dependence. Their acti- somatic motoneurones, raphe nuclei, and neurones of vation and inactivation kinetics are very fast. These cur- locus coeruleus and hypothalamus (Karschin et al., 2001; rents display an outward rectification that can be Talley et al., 2001). Conversely, TREK2, which is approximated by the Goldman–Hodgkin–Katz (GHK) restricted to the granule cell layer in the rodent cerebel- current equation that predicts a curvature of the I–V lum (Talley et al., 2001), is broadly expressed in human relationships in physiological asymmetric K+ conditions. brain, especially in the occipital lobe, putamen and thala- A notable property of TASK channels is their extreme mus (Lesage et al., 2000). Finally, a recent study showed sensitivity to variations in external pH in a narrow an abundant expression of the TASK2 protein in rat physiological range (TASK standing for TWIK-related brain (Gabriel et al., 2002), whereas the messenger for Acid-Sensitive K+ channel). They are inhibited by extra- this channel was barely detected by PCR in human and cellular acidosis with a midpoint of inhibition of 7.3 for mouse brains (Reyes et al., 1998; Medhurst et al., 2001).
Details
-
File Typepdf
-
Upload Time-
-
Content LanguagesEnglish
-
Upload UserAnonymous/Not logged-in
-
File Pages7 Page
-
File Size-