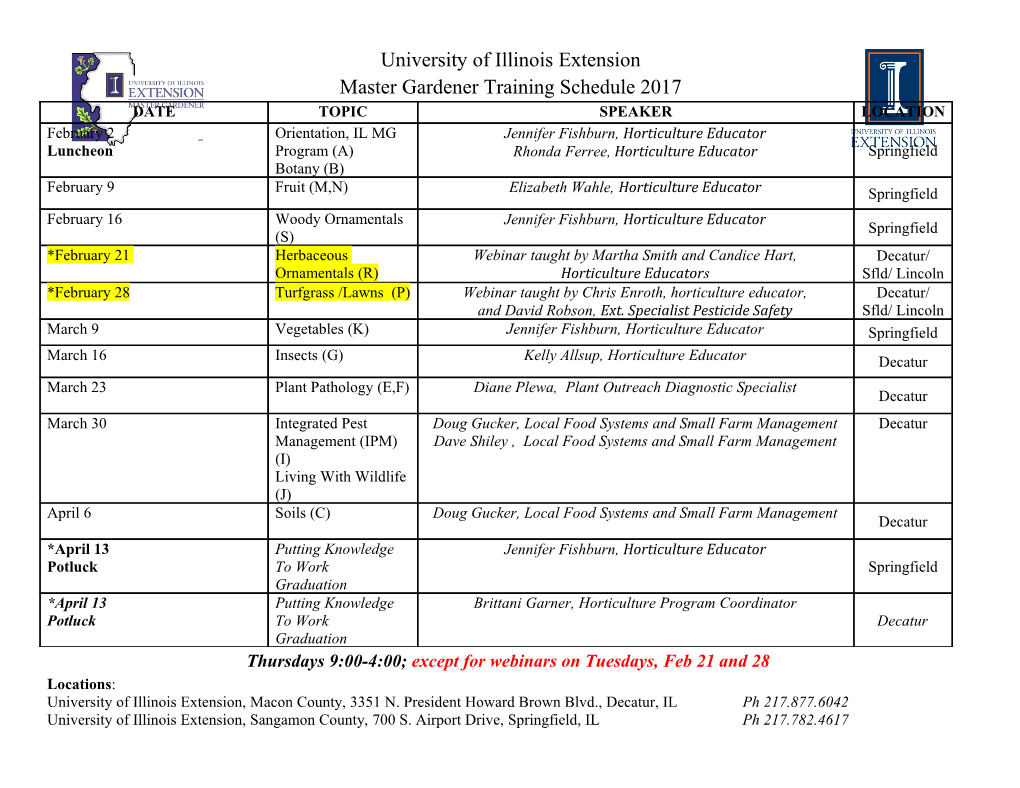
fJ"*! CARBONYL COMPOUNDS ALDEHYDES AND KETONES. ON REACT OF THE CARBONYL GROUP \ The carbonyl group, /C=O is a structural feature of many different types of compounds. It is present in carbon dioxide and in methanal, which represent respectively the high and low extremes in the level of oxidation of a carbonyl carbon: carbon dioxide methanal In between, there are carbonyl compounds ranging from aldehydes and ketones to carboxylic acids and their derivatives (esters, amides, anhydrides, and acyl 672 16 Carbonyl Compounds I. Aldehydes and Ketones. Addition Reactions of the Carbonyl Group halides). The naming of these compounds is described in Sections 7-4 to 7-7. R R R R \ \ \ \ /"=" /"=" /"=" /"=" H R HO RO aldehyde ketone carboxylic acid carboxylic ester R carboxyl ic X = F, CI, Br, I amide anhydride acyl halide At the upper end of the oxidation scale, along with CO,, are the carbonic acid derivatives such as carbonic esters, amides, halides, and carbonate salts, and isocyanates: carbonic ester carbonate bicarbonate carbonyl chloride (phosgene) carbonyl carbamic ester isocyanate diamide (urea) In this and succeeding chapters we describe the chemistry of these compounds with the intent of emphasizing the similarities that exist between them. The differences turn out to be more in degree than in kind. Even so, it is convenient to discuss aldehydes and ketones separately from carboxylic acids and, follow- ing some general observations about the carbonyl group, this chapter mainly is concerned with aldehydes and ketones. Apart from CO, and metal carbonates, the most abundant carbonyl compounds of natural origin are carboxylic esters and amides. These occur as fats and lipids, which are esters of long-chain alkanoic acids (pp. 789-791), and as proteins, which are polyamides of natural amino acids. The same struc- 16-1A The Carbonyl Bond Compared with Carbon-Carbon Double Bonds tural features are found in certain synthetic polymers, in particular the poly- esters (e.g., Dacron) and the polyamides (e.g., nylon 6): Dacron (polyester) nylon 6 (polyamide) lI Compared to carboxylic and carbonic acid derivatives, the less highly oxidized carbonyl compounds such as aldehydes and ketones are not so wide- spread in nature. That is not to say that they are unimportant. To the contrary. Aldehydes and ketones are of great importance both in biological chemistry and in synthetic organic chemistry. However, the high reactivity of the car- bony1 group in these compounds enables them to function more as intermedi- ates in metabolism or in synthesis than as end products. This fact will become evident as we discuss the chemistry of aldehydes and ketones. Especially important are the addition reactions of carbonyl groups, and this chapter is mostly concerned with this kind of reaction of aldehydes and ketones. 16-1 THE CARBONYL BOND 16-1A Comparison with Carbon-Carbon Double Bonds The carbonyl bond is both a strong bond and a reactive bond. The bond energy varies widely with structure, as we can see from the carbonyl bond energies in Table 16- 1. Methanal has the weakest bond (166 kcal) and carbon monoxide the strongest (257.3 kcal). Irrespective of these variations, the carbonyl bond not only is significantly stronger but also is more reactive than a carbon- carbon double bond. A typical difference in stability and-reactivity is seen in hydration: 674 16 Carbonyl Compounds I. Aldehydes and Ketones. Addition Reactions of the Carbonyl Group Table 16-1 Carbonyl Bond Energies Bond energy Compounds (kcal mole-') 0 0 :o=c:+--+:O--c: 257.3 O=C=O 192.0" H2C=0 166.0 H2C=C=0 184.8 RCH=O (aldehydes) 176 R2C=0 (ketones) 179 \ / C=C (a1 kenes) 146 / \ "Average of AH0 for breaking both of the C=O bonds. The equilibrium constant for ethene hydration is considerably greater than for methanal hydration, largely because the carbon-carbon double bond is weaker. Even so, methanal adds water rapidly and reversibly at room temperature without need for a catalyst. The corresponding addition of water to ethene occurs only in the presence of strongly acidic catalysts (Section 10-3E, Table 15-2). 16-1 B Structure and Reactivity The reactivity of the carbonyl bond is primarily due to the difference in elec- tronegativity between carbon and oxygen, which leads to a considerable con- tribution of the dipolar resonance form with oxygen negative and carbon positive: In terms of an atomic-orbital description, the carbonyl bond can be represented as shown in Figure 16-1. The carbon is sp2-hybridized so that its cr bonds (one of which is to oxygen) lie in one plane. The remaining p orbital on carbon is utilized to form a 7~ bond to oxygen. The polarity of the carbon- 16-1B Structure and Reactivity Figure 16-1 Atomic-orbital description of the carbonyl group. The CT bonds to carbon are coplanar, at angles near to 120"; the two pairs of unshared electrons on oxygen are shown as occupying orbitals n. oxygen double bond implies that the electrons of the 71- bond (and also the cr bond) are associated more with oxygen than with carbon. This is supported by the dipole moments1 of aldehydes and ketones, which indicate the degree of the polarization of the C=O bonds; the dipole moments are in the neigh- borhood of 2.7 D, which corresponds to 40-50% ionic character for the car- bony1 bond. Exercise 16-1 Draw valence-bond structures and an atomic-orbital model for carbon monoxide. Why can the bond energy of this molecule be expected to be higher than for other carbonyl compounds (see Table 16-I)? Explain why the dipole moment of CO is very small (0.13 debye).. lAn electrical dipole results when unlike charges are separated. The magnitude of the dipole, its dipole moment, is given by e x r, where e is the magnitude of the charges and r is the distance the charges are separated. Molecular dipole moments are measured in 0 0 debye units (D). A pair of ions, C and 0, as point charges at the C=O distance of 1.22 A, would have a dipole moment of 5.9 D. Thus, if the dipole moment of a carbonyl compound is 2.7 D, we can estimate the "% ionic character" of the bond to be (2.715.9) x 100 = 46%. The analysis is oversimplified in that the charges on the atom are not point charges and we have assumed that all of the ionic character of the molecule is associated with the C=O bond. One should be cautious in interpreting dipole moments in terms of the ionic character of bonds. Carbon dioxide has no dipole moment, but certainly has polar C=O bonds. The problem is that the dipoles associated with the C=O bonds of CO, are equal and opposite in direction to each other and, as a result, 60 260 60 cancel. Thus, 0-C-0 has no net dipole moment, even though it has highly po- lar bonds. 676 16 Carbonyl Compounds I. Aldehydes and Ketones. Addition Reactions of the Carbonyl Group Exercise 16-2 Which of the following compounds would you expect to have zero or nearly zero dipole moments? Give your reasoning and don't forget possible con- formational equilibria. (Models will be helpful.) The polarity of the carbonyl bond facilitates addition of water and other polar reagents relative to addition of the same reagents to alkene double bonds. This we have seen previously in the addition of organometallic compounds 60 60 60 60 R-MgX and R-Li to carbonyl compounds (Section 14-12A). Alkene double bonds are normally untouched by these reagents: Likewise, alcohols add readily to carbonyl compounds, as described in Sec- tion 15-4E. However, we must keep in mind the possibility that, whereas additions to carbonyl groups may be rapid, the equilibrium constants may be small because of the strength of the carbonyl bond. Exercise 16-3 The foregoing discussion explicitly refers to addition of polar reagents to carbonyl groups. Therefore an ionic mechanism is implied. Consider whether the same reactivity differences would be expected for ethene and methanal in the radical- chain addition of hydrogen bromide to methanal and ethene initiated by peroxides. What about the relative equilibrium constants? Show your reasoning. (Review Section 10-7.) H2C=0 + HBr - BrCH2-OH H2C=CH2 + HBr - BrCH2-CH, 16-1C Further Considerations of Reactivity 677 16-1C Further Considerations of Reactivity The important reactions of carbonyl groups characteristically involve addition at one step or another. For the reactions of organometallic reagents and al- cohols with carbonyl compounds (Chapters 14 and 15), you may recall that steric hindrance plays an important role in determining the ratio between addi- tion and other, competing reactions. Similar effects are observed in a wide variety of other reactions. We expect the reactivity of carbonyl groups in addition processes to be influenced by the size of the substituents thereon, because when addition occurs the substituent groups are pushed back closer to one another. In fact, reactivity and equilibrium constant decrease with increasing bulkiness of substituents, as in the following series (also see Table 15-3): Strain effects also contribute to reactivity of cyclic carbonyl compounds. The normal bond angles around a carbonyl group are about 120": Consequently if the carbonyl group is on a small carbocyclic ring, there will be substantial angle strain and this will amount to about 120" - 60" = 60" of strain for cyclopropanone, and 120" - 90" = 30" of strain for cyclobutanone (both values being for the L C-C-C at the carbonyl group). Addition of a nucleophile such as CH,OH (cf. Section 15-4E) to these carbonyl bonds creates a tetrahedral center with less strain in the ring bonds to C1: 678 16 Carbonyl Compounds I.
Details
-
File Typepdf
-
Upload Time-
-
Content LanguagesEnglish
-
Upload UserAnonymous/Not logged-in
-
File Pages64 Page
-
File Size-