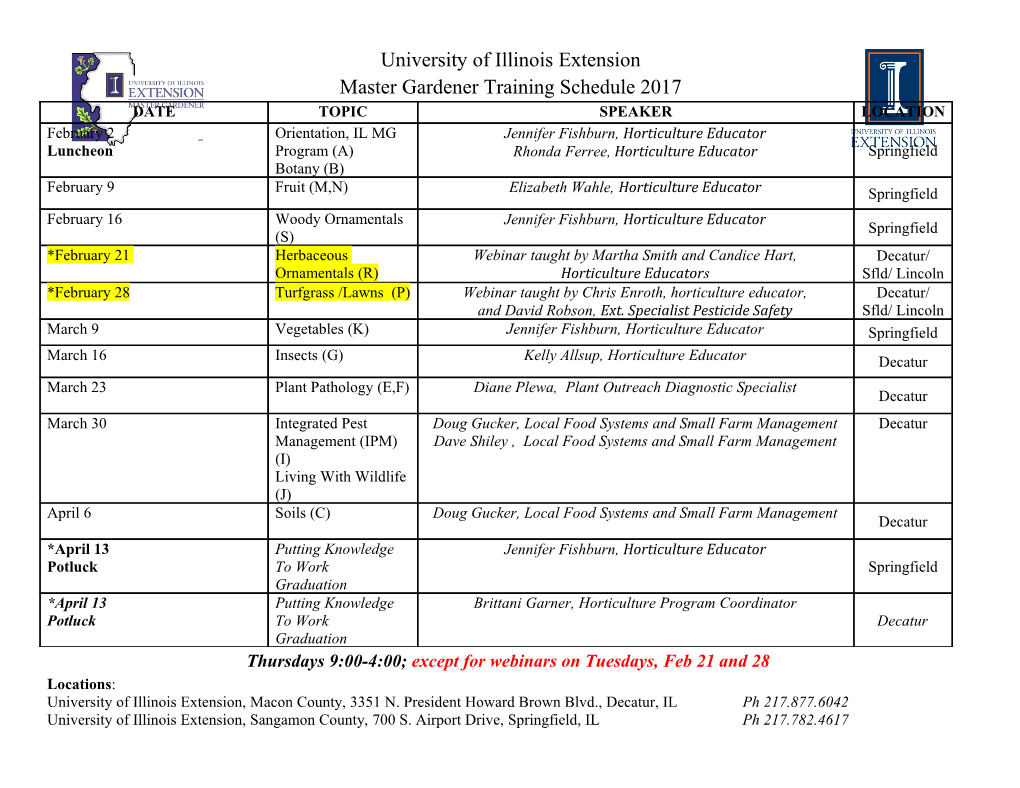
www.nature.com/bjc REVIEW ARTICLE Engaging chromatin: PRC2 structure meets function Paul Chammas1, Ivano Mocavini1 and Luciano Di Croce1,2,3 Polycomb repressive complex 2 (PRC2) is a key epigenetic multiprotein complex involved in the regulation of gene expression in metazoans. PRC2 is formed by a tetrameric core that endows the complex with histone methyltransferase activity, allowing it to mono-, di- and tri-methylate histone H3 on lysine 27 (H3K27me1/2/3); H3K27me3 is a hallmark of facultative heterochromatin. The core complex of PRC2 is bound by several associated factors that are responsible for modulating its targeting specificity and enzymatic activity. Depletion and/or mutation of the subunits of this complex can result in severe developmental defects, or even lethality. Furthermore, mutations of these proteins in somatic cells can be drivers of tumorigenesis, by altering the transcriptional regulation of key tumour suppressors or oncogenes. In this review, we present the latest results from structural studies that have characterised PRC2 composition and function. We compare this information with data and literature for both gain-of function and loss-of-function missense mutations in cancers to provide an overview of the impact of these mutations on PRC2 activity. British Journal of Cancer (2020) 122:315–328; https://doi.org/10.1038/s41416-019-0615-2 BACKGROUND and embryonic ectoderm development (EED) (Table 1). These Transcriptional diversity is one of the hallmarks of cellular three proteins form the minimal core that confers histone identity. It is largely regulated at the level of chromatin, where methyltransferase (HMT) activity. A fourth factor, retinoblastoma- different protein complexes act as initiators, enhancers and/or binding protein (RBBP)4/7 (also known as RBAP48/46), has a repressors of transcription. Among these complexes, are slightly lower stoichiometry and is dispensable for the enzymatic epigenetic modifiers, which are able to catalyse post- activity of the complex.22,23 Although there is very little diversity translational modifications (PTMs)—such as methylation, acet- in PRC2 core components, a large number of facultative subunits ylation, phosphorylation or ubiquitination—of histone proteins. have been shown to bind PRC2 in a sub-stoichiometric and cell- These modifications can influence gene expression by modulat- type specific manner,24–26 adding both to the complexity of ing chromatin accessibility, its interaction with other proteins recruitment of this complex to chromatin and to additional and its three-dimensional organisation. The Polycomb group possibilities of regulation of its enzymatic activity.27 Studies (PcG) proteins form histone-modifying complexes whose activity carried out over the past 5 years have shown that many of these is associated with transcriptional silencing of facultative hetero- facultative subunits bind in a mutually exclusive manner, giving chromatin.1–4 Two catalytically distinct complexes can be rise to two versions of the PRC2 complex. The first variant distinguished: the Polycomb repressive complex (PRC) 1, and (PRC2.1) comprises one of three Polycomb-like (PCL) proteins PRC2. PRC1 catalyses the mono-ubiquitination of lysine 119 on (PCL1/2/3, also named PHF1, MTF2 and PHF19, respectively) histone H2A (H2AK119ub),5,6 whereas PRC2 catalyses the mono-, as well as Elongin BC and Polycomb repressive complex di- and tri-methylation of lysine 27 on the histone H3 tail 2-associated protein (EPOP) or PRC2-associated LCOR isoform 1 (H3K27me1/2/3).7 In mice and humans, PRC2 is essential for (PALI1/2), while the other variant (PRC2.2) comprises Jumonji proper embryonic stem cell (ESC) fate specification, as it and AT-rich interaction domain 2 (JARID2) and adipocyte regulates the expression of key developmental genes.8–10 enhancer-binding protein 2 (AEBP2),26,28–30 in addition to the Indeed, depletion of PRC2 subunits leads to severe develop- core components. mental defects with early embryonic or perinatal lethality.10–17 Along with the interest in characterising the functional role of Mutations and/or dysregulation of PcG genes are found in accessory factors in regulating PRC2 activity, effort has also been several cancer types, especially haematological ones,18,19 as put into trying to gain structural insights into the complexity of well as in rare genetic diseases associated with overgrowth, such PRC2 and its subtypes. In this review, we discuss the latest findings as Weaver syndrome.20 These alterations can affect PRC2 regarding the PRC2 structure, focusing on the aspects that define recruitment and enzymatic activity, leading to changes in the the formation of different complex subtypes, its chromatin expression of tumour suppressors or oncogenes. targeting and its enzymatic activity. Finally, building on all the The PRC2 core comprises three stoichiometric factors: enhan- current structural knowledge, we highlight the potential effect of cer of Zeste (EZH)1 or EZH2, which has a SET domain and is the PRC2 mutations on complex integrity and activity, and the role of catalytic subunit of the complex;7,21 suppressor of Zeste (SUZ) 12; mutations of PRC2 components in cancer. 1Centre for Genomic Regulation (CRG), The Barcelona Institute of Science and Technology (BIST), Dr. Aiguader 88, Barcelona 08003, Spain; 2Universitat Pompeu Fabra (UPF), Barcelona, Spain and 3ICREA, Pg Lluis Companys 23, Barcelona 08010, Spain Correspondence: Luciano Di Croce ([email protected]) These authors contributed equally: Paul Chammas, Ivano Mocavini. Received: 14 June 2019 Accepted: 24 September 2019 Published online: 11 November 2019 © The Author(s), under exclusive licence to Cancer Research UK 2019 Engaging chromatin: PRC2 structure meets function P Chammas et al. 316 Table 1. Domain composition of PRC2 subunits Table 1 continued Protein Name Acronym Protein Name Acronym PHF19/PCL3 Tudor domain Tudor SUZ12 Zn finger binding domain ZnB PHD Domain PHD1 WD-domain binding 1 WDB1 PHD Domain PHD2 C2 domain C2 Extended Homology domain EH Zn Finger Zn Chromo domain Chromo WD-domain binding 2 WDB2 EPOP ELOBC binding box BC box VRN2-EMF2-FIS2-Su(z)12 box VEFS C-terminal region CTR EZH2 SANT1L-binding domain SBD AEBP2 Zn finger Zn1 EED-binding domain EBD Zn finger Zn2 β-addition motif BAM Zn finger Zn3 SET activation loop SAL Lysine/Arginine-rich domain KR stimulation-responsive motif SRM C2 binding domain C2B Swi3, Ada2, N-CoR and TFIIIB DNA- SANT1 binding domain 1 like H3K4 displacement domain H3K4D Motif connecting SANT1 and SANT2 MCSS JARID2 Transrepression domain TR SANT2-like SANT2 Ezh1/2-binding domain CXC domain CXC Nucleosome interaction domain Su(var)3-9, E(z) and Trx domain SET Jumonji N-term JmjN Post-SET Post-SET AT-rich interaction domain ARID EED WD-repeat region WD1 Jumonji C-term JmjC WD2 Zinc finger ZF 1234567890();,: WD3 WD4 WD5 STRUCTURAL BASIS FOR PRC2 COMPLEX FORMATION AND WD6 FUNCTION The association of the trimeric core (EZH2, SUZ12 and EED) with WD7 RBBP4 results in a stable, four-lobed structure (Fig. 1)27,28 that PALI1 Nuclear receptor binding box NR comes together to mediate HMT activity. CTBP binding motifs (x2) CTBP G9A interaction region The catalytic lobe Pali interaction with PRC2 domain PIP The C-terminal region of EZH2, comprising the CXC domain (a cysteine-rich region) and the SET domain, forms the catalytic RBBP4 WD-repeat region WD1 lobe, in which the HMT activity of PRC2 resides (Fig. 1e). The WD2 active site presents two pockets in the SET domain: the first one WD3 is a highly hydrophobic channel (Y641, F667, F724, Y726 and WD4 Y728), which accommodates the long aliphatic chain of the WD5 lysine substrate (Fig. 1c). The end of this channel is connected to a second pocket, in which the cofactor S-adenosyl methionine WD6 (SAM) is positioned in an orientation that brings its methyl WD7 group in close proximity to the ε-amino group of the lysine. RBBP7 WD-repeat region WD1 Residues that lie at the interface of these two pockets (e.g. Y641, WD2 A677 and A687) are crucial for catalysis, and their mutation fi WD3 results in changes in af nity for the substrate that are associated with gain-of-function phenotypes (as discussed below). The WD4 assembly of the trimeric core is essential for HMT activity: in WD5 isolation, EZH2 adopts an autoinhibited conformation, with the WD6 post-SET domain (the region C-terminal to the SET domain) WD7 folded upwards into the lysine-binding cleft, blocking the 31–33 PHF1/PCL1 Tudor domain Tudor substrate from engaging the active site. This mechanism, which seems to be conserved in the H3K9 methyltransferase PHD Domain PHD1 Suv39h2,34 might provide a ‘safety catch’ against spurious PHD Domain PHD2 histone methylation. Extended Homology domain EH Chromo domain Chromo The regulatory lobe MTF2/PCL2 Tudor domain Tudor The catalytic lobe is in close contact with the regulatory lobe, which is formed by the association of EED with the N-terminal PHD Domain PHD1 domain of EZH2 (Fig. 1d). The long α-helix of the EED-binding PHD Domain PHD2 domain (EBD) and the β-addition motif (BAM) of EZH2 wrap Extended Homology domain EH around the bottom (or closed end) and side, respectively, of the 35,36 Chromo domain Chromo WD-repeat seven-bladed β-propeller of EED. This conforma- tion is necessary to maintain EED in a stable position while leaving Engaging chromatin: PRC2 structure meets function P Chammas et al. 317 Middle lobe a EZH2EZH2 b c H3 SETSET K27 Y728 SANT2 F667 L616 F686 Y726 SUZ12 MCSS F724 VEFS Y641 M110 A687 EZH2EZH2 F665 F566 SETSET W624 A677 SAM VEFS P618 EZH2EZH2 R685 SALSAL V107 P108 180° Regulatory lobe Catalytic lobe d EZH2 e SANT1 SBD EED CXC EBD SRM 90° SET BAM SUZ12 RBBP4 Docking lobe f SUZ12 g h JARID2 Zn/ZnB TR L87 E91 E84 ZnB R164 L161 F86 WDB2 F160 Zn R98 F157 F90 T159 JARID2 L158 TR F432 W452 SUZ12 Zn/ZnB P451 Y430 D156 C2 WDB1 E155 R445 Fig.
Details
-
File Typepdf
-
Upload Time-
-
Content LanguagesEnglish
-
Upload UserAnonymous/Not logged-in
-
File Pages14 Page
-
File Size-