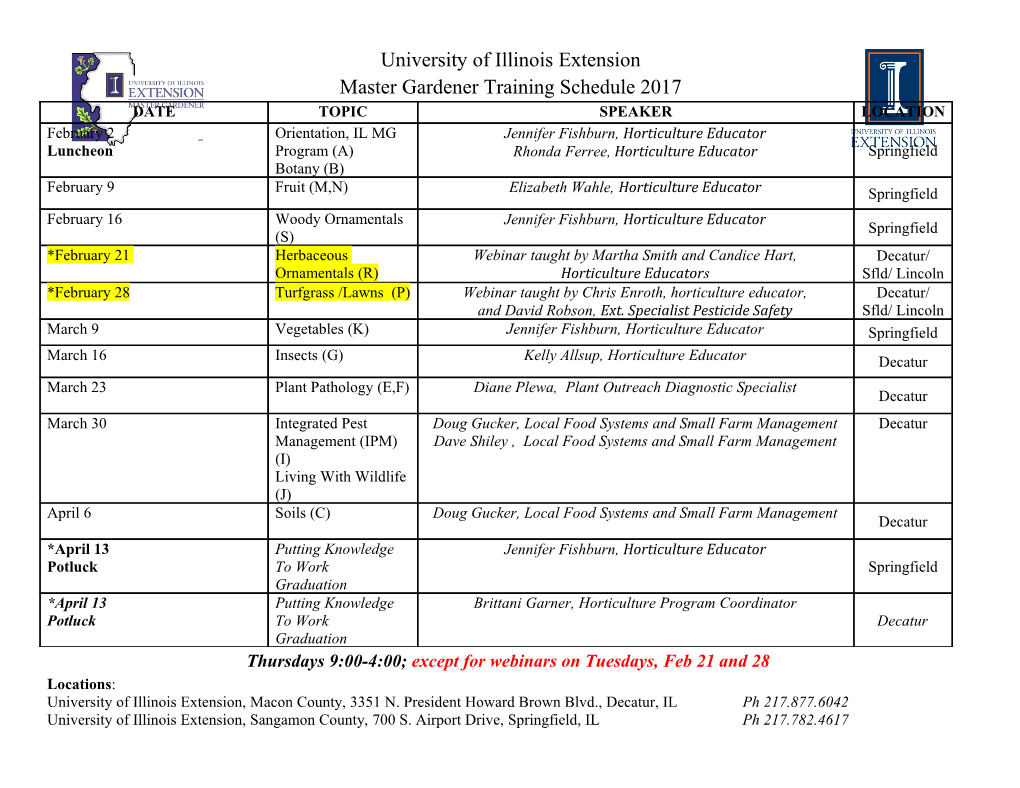
Sibson Earth, Planets and Space (2017) 69:113 DOI 10.1186/s40623-017-0699-y FULL PAPER Open Access Tensile overpressure compartments on low‑angle thrust faults Richard H. Sibson* Abstract Hydrothermal extension veins form by hydraulic fracturing under triaxial stress (principal compressive stresses, σ1 > σ2 > σ3) when the pore-fuid pressure, Pf, exceeds the least compressive stress by the rock’s tensile strength. Such veins form perpendicular to σ3, their incremental precipitation from hydrothermal fuid often refected in ‘crack- seal’ textures, demonstrating that the tensile overpressure state, σ3′ (σ3 Pf) < 0, was repeatedly met. Systematic arrays of extension veins develop locally in both sub-metamorphic and= metamorphic− assemblages defning tensile overpressure compartments where at some time Pf > σ3. In compressional regimes (σv σ3), subhorizontal extension veins may develop over vertical intervals <1 km or so below low-permeability sealing= horizons with tensile strengths 10 < To < 20 MPa. This is borne out by natural vein arrays. For a low-angle thrust, the vertical interval where the tensile overpressure state obtains may continue down-dip over distances of several kilometres in some instances. The over- pressure condition for hydraulic fracturing is comparable to that needed for frictional reshear of a thrust fault lying close to the maximum compression, σ1. Under these circumstances, especially where the shear zone material has varying competence (tensile strength), afecting the failure mode, dilatant fault–fracture mesh structures may develop throughout a tabular rock volume. Evidence for the existence of fault–fracture meshes around low-angle thrusts comes from exhumed ancient structures and from active structures. In the case of megathrust ruptures along sub- duction interfaces, force balance analyses, lack of evidence for shear heating, and evidence of total shear stress release during earthquakes suggest the interfaces are extremely weak (τ < 40 MPa), consistent with weakening by near- lithostatically overpressured fuids. Portions of the subduction interface, especially towards the down-dip termination of the seismogenic megathrust, are prone to episodes of slow-slip, non-volcanic tremor, low-frequency earthquakes, very-low-frequency earthquakes, etc., attributable to the activation of tabular fault–fracture meshes at low σ3′ around the thrust interface. Containment of near-lithostatic overpressures in such settings is precarious, fuid loss curtailing mesh activity. Introduction σv. Normal faulting prevails where the crust is under Material properties aside, the formation and reactiva- extension with σv = σ1; strike-slip faulting occurs where tion of brittle faults and fractures is largely governed by σv = σ2; and thrust faulting develops where the crust is the triaxial stress state within the rock mass and by the shortening under horizontal compression with σv = σ3. pore-fuid pressure, Pf, within the rock mass (Hubbert Information from borehole measurements (Townend and Rubey 1959; Jaeger and Cook 1979). Recognizing the and Zoback 2001), earthquake focal mechanisms (Célé- boundary condition of zero shear stress along the Earth’s rier 2008), and palaeostress inversions (Lisle et al. 2006) free surface (taken as horizontal), Anderson (1905) pos- demonstrates that ‘Andersonian’ stress states (one princi- tulated the existence of three basic stress regimes in the pal stress vertical and the other two horizontal) prevail crust depending which of the principal compressive over large areas of continental and oceanic crust (Zoback stresses (σ1 > σ2 > σ3) coincides with the vertical stress, 1992). Tese ‘Andersonian’ stress orientations provide useful reference states for general consideration, though signifcant departures undoubtedly occur. For exam- *Correspondence: [email protected] Department of Geology, University of Otago, Dunedin 9054, New Zealand ple, although a subduction interface shear zone (SISZ) © The Author(s) 2017. This article is distributed under the terms of the Creative Commons Attribution 4.0 International License (http://creativecommons.org/licenses/by/4.0/), which permits unrestricted use, distribution, and reproduction in any medium, provided you give appropriate credit to the original author(s) and the source, provide a link to the Creative Commons license, and indicate if changes were made. Sibson Earth, Planets and Space (2017) 69:113 Page 2 of 15 is, in essence, a large-scale thrust fault accommodating Controls on brittle failure of rock underthrusting of oceanic lithosphere, signifcant depar- Criteria for all modes of brittle failure in rock include tures from vertical and horizontal stress trajectories are the levels of diferential stress and pore-fuid pressure ′ expected from: (1) the kinematic control exerted by a [through the principle of efective stress, σn = (σn − Pf)]. weak plate boundary shear zone; (2) force balance anal- Te two drivers to failure, therefore, are (σ1 − σ3) and Pf, yses associated with a tapering accretionary wedge (e.g. which may act separately or together. In ‘classical’ rock Dahlen 1990); and (3) time variations in the stress feld mechanics (e.g. Secor 1965; Jaeger and Cook 1979; Sib- as a consequence of stress cycling associated with inter- son 2000), the composite Grifth–Coulomb failure curve ′ mittent megathrust rupturing along the SISZ (Hasegawa for intact rock in τ/σn space can be normalized to nomi- et al. 2012). nal rock tensile strength, To (half the cohesive strength). Hubbert and Rubey (1959) were the frst to apply the Te ratio of diferential stress to rock tensile strength simple principle of efective stress to rocks whereby (σ1 − σ3)/To then determines the potential mode of brit- pore-fuid pressure reduces all normal stresses to ‘efec- tle failure (Fig. 1). For ‘generic’ internal friction, μi 0.75 ′ = tive’ values, σn = (σn − Pf), suggesting also that fuid in the compressional feld, extension fractures form only overpressures were a means of reducing basal fric- when (σ1 − σ3)/To < 4, hybrid extensional-shear fractures tion allowing emplacement of thrust sheets along low- require 4 < (σ1 − σ3)/To < 5.66, and compressional shear angle overthrusts. Tey also defned a pore-fuid factor, fractures (faults) form when (σ1 − σ3)/To > 5.66 (Secor λv = Pf/σv, usefully relating the level of pore-fuid pres- 1965). Additionally, as noted above, the presence of exist- sure with respect to the vertical stress. Where fuid ing fractures appropriately oriented for reshear within pressure, Pf, in pore and/or fracture space is freely inter- the stress feld suppresses the formation of any new connected up to a water table at the earth’s surface, the extensional or hybrid extensional-shear fractures. Fluid pore-fuid pressure is hydrostatic (λv ~ 0.4). Pore-fuids circulation through a fractured rock mass may, how- are overpressured wherever pore-fuid pressures exceed ever, restore cohesive and tensile strength by hydrother- hydrostatic values (i.e. λv > 0.4). Fluid at a depth, z, is mal cementation (e.g. silicifcation), efectively restoring overpressured towards lithostatic values (λv → 1.0) when ‘intact’ character. Pf approaches the lithostatic load (σv = ρgz, where ρ is Hydraulic extension fracturing can thus only be average rock density and g the gravitational acceleration). induced: (a) within intact rock in the absence of cohe- Supralithostatic overpressures (λv > 1.0) occur where sionless faults suitably oriented for shear reactivation; (b) Pf > σv. in settings where existing fractures are severely misori- Formation and opening of extension fractures per- ented for reshear (containing the σ2 axis and lying at >c. pendicular to least compressive stress, σ3, in intact rock 60° to σ1); and (c) where existing fractures have regained occurs by hydraulic fracturing when cohesive strength through hydrothermal cementation, Pf = σ3 + To (1) etc. Rock tensile strength is thus critical in determining whether rock fails in tension or in shear under increased where To is rock tensile strength, provided (σ1 − σ3) < 4 fuid pressure. Under the same diferential stress, fuid- To inhibiting shear failure of the rock (Secor 1965; Ether- induced failure in heterogeneous material of mixed com- idge 1983). For competent sedimentary rocks and crys- petence (difering tensile strength) may therefore give talline rocks with To ~ 10 MPa and ~20 MPa, respectively rise to mixed-mode brittle failure with volumetric fault– (Lockner 1995), diferential stresses are restricted to <40 fracture meshes comprising interlinked shear and exten- and <80 MPa. A local absence of existing fractures suit- sional fractures distributed throughout the rock mass ably oriented for shear reactivation is also a prerequi- (Sibson 1996, 2000). site. Te tensile overpressure state (Pf > σ3) thus defnes portions of the rock mass with the potential for hydrau- Tensile overpressure compartments lic fracturing leading to the formation of extension vein Extension veins [often with ‘crack-seal’ infllings of arrays. quartz, calcite, etc., refecting incremental precipita- In compressional settings where σv = σ3, the tensile tion (Ramsay 1980)] thus diagnose the tensile overpres- ′ overpressure state (Pf > σ3) equates to the maximum sure state (σ3 = (σ3 − Pf) < 0, or Pf > σ3) once having sustainable overpressure and is close to lithostatic (Sib- been achieved within the rock mass. In extensional son 2003). It is conducive to the development and fuid (σv = σ1) and strike-slip (σv = σ2) stress regimes where activation of dilatant
Details
-
File Typepdf
-
Upload Time-
-
Content LanguagesEnglish
-
Upload UserAnonymous/Not logged-in
-
File Pages15 Page
-
File Size-